Originally Authored by Csaba Juhasz, Christopher Lai, Michael E. Behen, Otto Muzik, Emily J. Helder, Diane C. Chugani, and Harry T. Chugani in the Archives of Neurology
Abstract
Objective: To assess the role of gray and white matter volume loss vs seizures in cognitive impairment of children with Sturge-Weber syndrome with unilateral involvement.
Design: Patients were enrolled in this prospective cohort during a period of 3 years.
Setting: Pediatric neurology clinic with national referral through the Sturge-Weber Foundation.
Participants: Twenty-one children (age range, 1 year 6 months to 10 years 4 months) with unilateral Sturge- Weber syndrome.
Main Outcome Measures: Cortical gray matter and hemispheric white matter volumes were measured on segmented volumetric magnetic resonance imaging and correlated with the age of the participants. Global intellectual function (IQ) was correlated with magnetic resonance imaging and seizure variables in both univariate and multivariate analyses.
Results: Both gray and white matter volumes showed an age-related linear increase. Tissue volumes on the side of the angioma showed a positive correlation with IQ after controlling for age in univariate regression analyses (white matter, r=0.71, P.001; gray matter, r=0.48, P=.03), while seizure variables did not correlate with IQ (P.1). A multivariate regression showed that hemispheric white matter volume ipsilateral to the angioma was an independent predictor of IQ (R=61, P=.006), which also showed a negative correlation with age (R=−0.52, P=.022) but no correlation with gray matter volumes.
Conclusions: Early hemispheric white matter loss may play a major role in cognitive impairment in children with Sturge-Weber syndrome. Future therapeutic approaches should aim at preserving white matter integrity in addition to seizure control to improve cognitive outcome.
Keywords:
Introduction
STURGE-WEBER SYNDROME (SWS) is a neurocutaneous disorder characterized by port wine stains, glaucoma, and pial angiomatosis involving 1 cerebral hemisphere in 85% of the cases.(1) Early white matter (WM) abnormalities (referred to as accelerated myelination) followed by both WM and gray matter (GM) volume loss have been reported in SWS,(2,3) but their pathomechanism and clinical correlates have not been clarified. Many affected children show progressive cognitive decline, which is commonly associated with intractable seizures.(4) However, seizures can be controlled with medication in about 40% to 50% of SWS patients(5) ; almost 50% of SWS patients with seizures are intellectually normal or only slightly delayed.(5,6) Thus, seizures during the course of the disease fail to provide sufficient prognostic information regarding the cognitive outcome in SWS. Current clinical practice in SWS focuses on preventing seizures by medication or resective epilepsy surgery. It is likely, however, that other factors not directly related to seizures, such as progression of the underlying pathology and resulting cell and tissue volume loss, contribute to neurological and cognitive abnormalities. The goal of the present study was to establish the relationship between global intellectual functioning and cortical GM and hemispheric WM volumes measured in segmented, volumetric magnetic resonance images (MRIs) in a prospectively collected group of children with unilateral SWS and seizures.
Methods
Subjects
Twenty-one children (13 girls; age range, 1 year 6 months to 10 years 4 months; mean age, 5.3 years) (Table) with SWS and unilateral hemispheric involvement and a history of partial seizures underwent MRI at the Magnetic Resonance Research Facility at Wayne State University. These children were selected from a cohort of 22 consecutive children with unilateral SWS who were recruited between July 2003 and June 2006 for a prospective neuroimaging research study approved by the Human Investigation Committee at Wayne State University. The children were recruited by the Pediatric Neurology Clinic of the Children’s Hospital of Michigan, which receives both regional and national patient referrals through the Sturge-Weber Foundation. One of the 22 children, a 3-year-old boy, was not included in our study because of an extensive hemispheric atrophy and calcifications that precluded reliable segmentation of GM and WM. Annual seizure frequency for the year before scanning was obtained from medical records and parental interviews. All but 3 children were on chronic antiepileptic medication, including monotherapy or polytherapy with oxcarbazepine (n=8), valproate semisodium (n=6), levetiracetam (n=6), carbamazepine (n=5), phenobarbital (n=2), and topiramate (n=1). All patients had undergone a neurological examination prior to the imaging studies. Written informed consent of the parent or legal guardian was obtained.
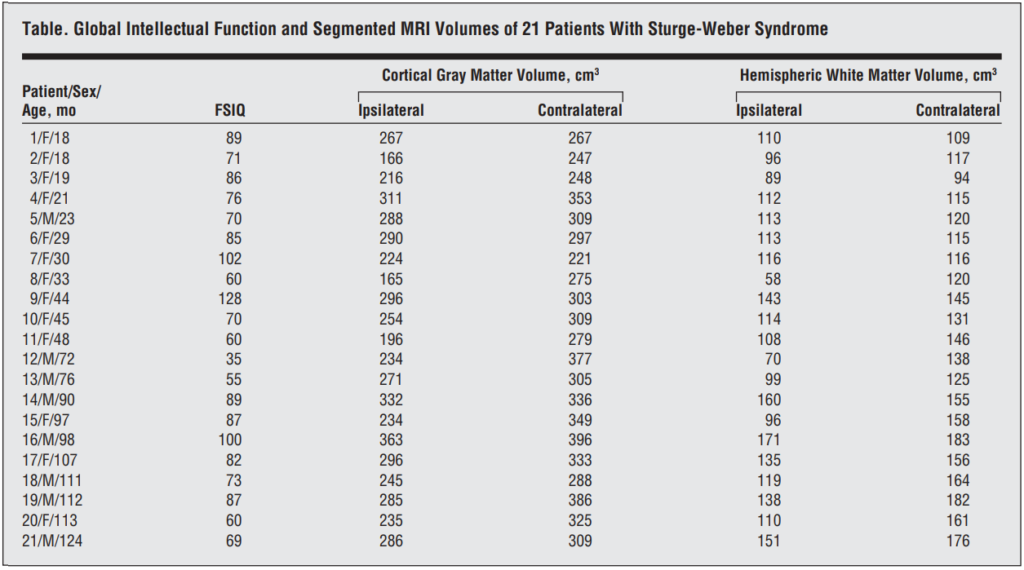
Assessment of cognitive functions
All children received a neuropsychological assessment within 1 day before the imaging studies. Global intellectual functions were assessed using age-appropriate tests (the Bayley Scales of Infant Development(7) [age range, 18-36 months]; Wechsler Preschool and Primary Scales of Intelligence, Third Edition [age range, 3-6 years]; and the Wechsler Intelligence Scales for Children, Third Edition [age, 6 years]),(8) which yielded the Mental Developmental Index (from the Bayley Scales of Infant Development) or the full-scale IQ (FSIQ; from the Wechsler Preschool and Primary Scales of Intelligence, Third Edition, and Wechsler Intelligence Scales for Children, Third Edition). The Mental Developmental Index is an excellent estimate of IQ and these indices are highly correlated (r0.8).(9) Therefore, we have used the term FSIQ in all children for simplicity. Verbal and nonverbal intellectual functions were available for a subgroup (n=15) of children (those who completed the Wechsler Preschool and Primary Scales of Intelligence, Third Edition, and/or the Wechsler Intelligence Scales for Children, Third Edition). Manual dexterity scores were also obtained in 17 children using age-appropriate tests (Purdue pegs and grooved pegs).
MRI data acquistion
All MRI studies were carried out on a MAGNETOM Sonata 1.5-T MRI scanner (Siemens, Erlangen, Germany) using a standard head coil. An axial 3-dimensional gradient-echo T1-weighted (repetition time/echo time, 20/5.6 milliseconds; flip angle, 25°; voxel size, 1 * 0.5 * 2 mm3 ) scan was acquired for volumetric measurements. A postgadolinium (0.1 mmol/kg) T1-weighted image was used to identify the leptomeningeal angioma. Children younger than 7 years of age were sedated with pentobarbital (3 mg/kg) followed by fentanyl citrate (1 µg/kg).
Image processing
All volumetric MRI volumes were processed using the SPM2 software package (Statistical Parametric Mapping; Wellcome Department of Cognitive Neurology, London, England; http: //www.fil.ion.ucl.ac.uk/spm).(10) A pediatric MRI template was created using images of all 21 children by applying a 7-parameter affine (linear) transformation (3 translation, 3 rotation, and 1 scaling parameter) to minimize the sum of squares between the individual image volume and the default MRI template. These transformed image volumes were then averaged to yield the study-specific pediatric MRI template. Subsequently, segmentation of MRI volumes was performed using the maximum likelihood mixture model.(11) Finally, all images were transformed back into native space using the inverse transformation matrix. As a result, 3 new image volumes in native space were created with voxel values between 0 and 1 representing GM, WM, and cerebrospinal fluid probabilities (Figure 1). All segmented images were inspected to ensure reasonable segmentation results. To derive cortical GM and hemispheric WM volumes, left and right hemispheric regions of interest were defined in all supratentorial image planes. All regions of interest were laid onto the probability images, and cortical GM and hemispheric WM volumes were calculated by multiplying the respective region of interest sum with the voxel volume for each hemisphere ipsilateral and contralateral to the angioma.
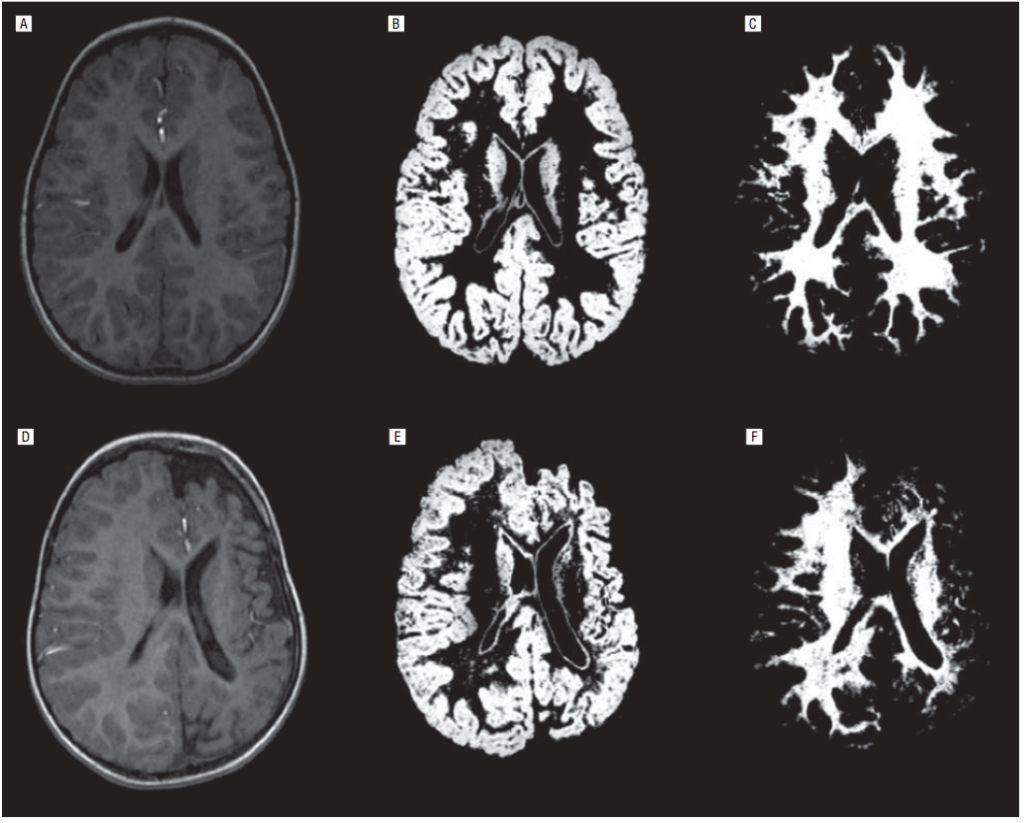
Statistical analysis
Initially, an F test was applied to determine whether age-related changes in GM and WM volume were linear or quadratic. To test whether changes of GM or WM volumes with age differed between the ipsilateral and contralateral side, a bootstrapping procedure was applied. To determine predictors of FSIQ, first, the effects of angioma side and sex on FSIQ values, as well as on GM and WM volumes, were evaluated using an analysis of variance with age as a covariate. Because frequent seizures as well as impaired motor functions could affect IQ scores, seizure variables (seizure frequency and duration, and age at onset of epilepsy) and manual dexterity scores of the dominant hand were correlated with IQ scores using Spearman rank correlations. Full-scale IQ and segmented tissue volumes were correlated by Pearson correlations with age as a covariate. Finally, significant predictors of IQ in the univariate analyses were entered into a multivariate regression analysis to determine the independent contribution of each variable to IQ. These regression analyses were repeated for cognitive subdomains (verbal and nonverbal IQ) in a subgroup of patients where such data were available. P=.05 was considered significant.
Results
Decrease GM and WM volumes on the side of the angioma
Both the ipsilateral cortical GM and hemispheric WM volumes were smaller as compared with the side contralateral to the angioma. For the cortical GM volume, the mean asymmetry between the 2 hemispheres was 18% (SD, 16%; mean volume difference, 50 cm3 ), whereas for the hemispheric WM volume, the mean asymmetry was 20% (SD, 21%; mean volume difference, 24 cm3 ).
Correlation between age and GM/WM volumes
Both the ipsilateral cortical GM and hemispheric WM volumes were smaller as compared with the side contralateral to the angioma. For the cortical GM volume, the mean asymmetry between the 2 hemispheres was 18% (SD, 16%; mean volume difference, 50 cm3 ), whereas for the hemispheric WM volume, the mean asymmetry was 20% (SD, 21%; mean volume difference, 24 cm3 ).
Relationship between cognitive functions, motor functions, and epilepsy, and GM/WM volumes
Full-scale IQ values, as well as GM and WM values (after controlling for age), were not different in patients with leftvs right-sided angioma (P.6 in all comparisons) or between sexes (P.2). Therefore, considering the limited number of patients, they were not separated based on sex or angioma side in the subsequent correlation analyses.
Seizure frequency during the year before MRI varied widely from no seizures (n=5) to approximately 400 seizures (n=1), with a median annual seizure frequency of 3 seizures. Eighteen of the 21 children had fewer than 1 seizure per month. Duration of epilepsy varied from 2 weeks to 116 months (median, 45 months), while age at onset was 3 to 29 months (median, 8 months). None of these seizure variables showed correlation with FSIQ values (P=.14-.31). None of the patients had severe hemiparesis on neurological examination. Manual dexterity scores showed significant impairment (SD, 2) in this domain in only 5 of the 17 children for the dominant hand (where such scores were available), and 8 children showed significant impairment for the nondominant hand. IQ values showed no correlation with manual dexterity scores of the dominant hand (FSIQ,r=−0.07, P=.80; verbal IQ, r=−0.01, P=.98; nonverbal IQ, r=0.26, P=.37). Altogether, these data indicated that neither seizures nor impaired motor functions were significant predictors of intellectual functions and therefore none of these clinical variables were entered into subsequent multivariate regression analyses.
Full-scale IQ showed a positive correlation with the ipsilateral WM volume (r=0.71, P.001) and ipsilateral cortical GM volume (r=0.48, P=.03) in univariate regression analyses, but not with contralateral tissue volumes (GM, r=−0.03, P=.88; WM, r=0.41, P=.07) after controlling for age. In a multivariate regression analysis, ipsilateral hemispheric WM volume showed a strong positive correlation with FSIQ (partial correlation, R=0.61, P=.006) (Figure 3), age showed a negative correlation with FSIQ (R=−0.52, P=.02), while cortical GM volume showed no correlation with FSIQ values (R=−0.13, P=.59) (Figure 3). The correlations with ipsilateral WM volumes were significant for both verbal IQ (R=0.56, P=.046) and nonverbal IQ (R=0.59, P=.035) in a subgroup of 15 children where such data were available.
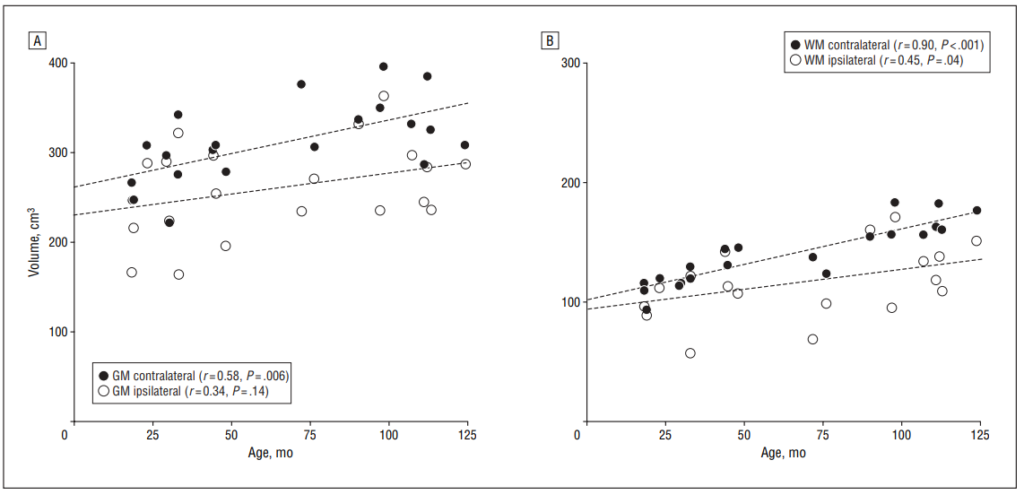
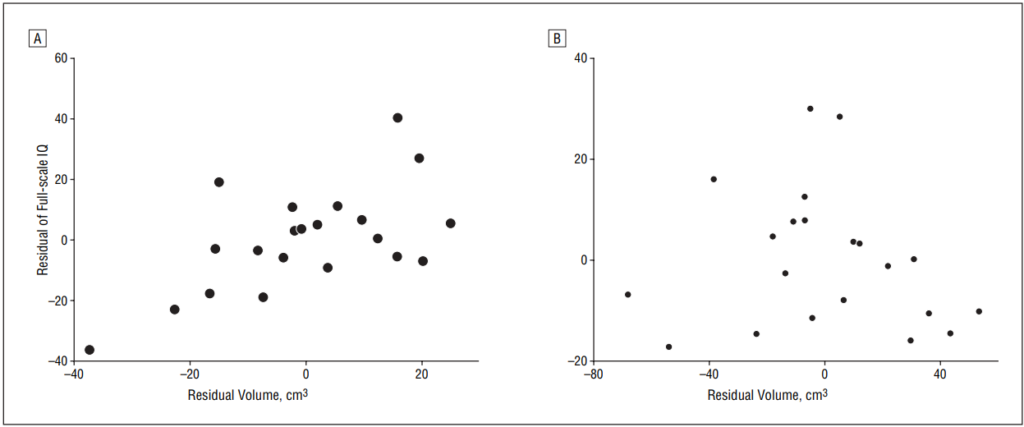
Comment
Despite the limited number of cases, the robust findings of our study indicate that WM volume in the affected hemisphere is indeed a major independent predictor of cognitive impairment in these children with SWS. A recent study reported a weak correlation between cortical volume asymmetry and cognitive function in SWS, but WM changes were not evaluated.(12) In healthy brains, intelligence is associated with both GM and WM volumes,(13) but specific cognitive functions are related to volumes of distinct GM regions.(14,15) Thus, GM volumes in specific brain regions, rather than total cortical GM volume in the entire hemisphere, may be better predictors of various aspects of cognitive functions. Anatomical reorganization in nonaffected regions(16) may also confound the association between GM volume and IQ. Magnetic resonance imaging studies in healthy children have shown that maturation of WM is an important process in cognitive development, probably owing to increased fiber organization and density.(17) Decreased WM volume following radiotherapy for brain tumors in children is associated with cognitive dysfunction,(18) and regional WM volumes were reported to be strong predictors of neurodevelopmental outcomes in preterm infants.(19) Our study provides evidence that abnormal development or loss of WM may be a critical factor of cognitive decline in SWS. Incomplete maturation or disruption of fiber tracts connecting various cortical and subcortical structures may lead to loss of functional connectivity, impaired efficiency of information processing, and abnormal cognitive development. Similar mechanisms of cognitive aging have been proposed based on diffusion tensor and magnetization transfer MRI studies.(20)
The mechanism most likely causing early WM abnormalities in patients with SWS is hypoxic injury due to an impaired venous drainage of the affected hemisphere, leading to an apparent accelerated myelination followed by WM atrophy.(2,3) Currently, there are no specific pharmacological approaches for preventing hypoxiainduced WM injury. Aspirin has been proposed to diminish neurological progression due to ischemia in SWS,(21) but no rigorous clinical trials have been performed to substantiate its potential benefit. Recent studies have revealed several important mechanisms of ischemic WM injury that could provide novel therapeutic targets. For example, N-methyl-D-aspartate receptors expressed on oligodendrocytes play a crucial role in ischemiainduced WM injury.(22) Another potential mechanism of such injury is related to the release of adenosine leading to A1 adenosine receptor activation, which may play a major role in periventricular WM damage in preterm infants following hypoxic-ischemic injury.(23) This damage can be diminished by adenosine antagonists.(24) Thus, novel therapeutic approaches, if administered early, may offset some of the detrimental effects of hypoxia on the developing WM, thus leading to improved cognitive outcome in SWS.
There are some methodological issues that should be considered when interpreting our results. Segmentation of tissue compartments may be inaccurate if a large proportion of voxels belongs to abnormal tissue types, such as calcified tissue. Several patients in the present cohort indeed showed focal areas of calcification on their previous computed tomographic scans, but none of them had extensive multilobar calcifications. To account for morphological differences between adult and pediatric brains, we have used a custom-made, study-specific, pediatric MRI template. This approach has been validated in previous developmental MRI studies of children with a wide age range.(25) We did not determine regional tissue volume changes and also did not assess sex effects or effects of antiepileptic medication, some of which could potentially influence cognitive functions but would be hard to control for considering the large variety and combinations of antiepileptic drugs. With continuing recruitment of more patients, the effects of regional GM and WM changes, lesion side, sex, and medication can be addressed in future studies.
References
1. Roach ES, Bodensteiner JB. Neurologic manifestations of Sturge-Weber syndrome. In: Bodensteiner JB, Roach ES, eds. Sturge-Weber Syndrome. Mt Freedom, NJ: Sturge-Weber Foundation; 1999:27-38.
2. Adamsbaum C, Pinton F, Rolland Y, Chiron C, Dulac O, Kalifa G. Accelerated myelination in early Sturge-Weber syndrome: MRI-SPECT correlations. Pediatr Radiol. 1996;26(11):759-762.
3. Pfund Z, Kagawa K, Juhasz C, et al. Quantitative analysis of gray- and whitematter volumes and glucose metabolism in Sturge-Weber syndrome. J Child Neurol. 2003;18(2):119-126.
4. Kramer U, Kahana E, Shorer Z, Ben-Zeev B. Outcome of infants with unilateral Sturge-Weber syndrome and early onset seizures. Dev Med Child Neurol. 2000; 42(11):756-759.
5. Sujansky E, Conradi S. Outcome of Sturge-Weber syndrome in 52 adults. Am J Med Genet. 1995;57(1):35-45.
6. Aicardi J, Arzimanoglou A. Sturge-Weber syndrome. Int Pediatr. 1991;6:129-134.
7. Bayley N. Bayley Scales of Infant Development. 2nd ed. San Antonio, TX: Psychological Corp, Harcourt Brace & Co; 1993.
8. Wechsler D. Wechsler Intelligence Scale for Children. 3rd ed. San Antonio, TX: Psychological Corp; 1991.
9. Wechsler D. WPPSI-III: Technical and Interpretive Manual. San Antonio, TX: Psychological Corp; 2002.
10. Worsley KJ, Marrett S, Neelin P, et al. A unified statistical approach for determining significant signals in images of cerebral activation. Hum Brain Mapp. 1996; 4(1):58-73.
11. Hartigan JA. Clustering Algorithms. New York, NY: John Wiley & Sons; 1975: 113-129.
12. Kelley TM, Hatfield LA, Lin DD, Comi AM. Quantitative analysis of cerebral cortical atrophy and correlation with clinical severity in unilateral Sturge-Weber syndrome. J Child Neurol. 2005;20(11):867-870.
13. Posthuma D, De Geus EJ, Baare´ WF, Hulshoff , Pol HE, Kahn RS, Boomsma DI. The association between brain volume and intelligence is of genetic origin. Nat Neurosci. 2002;5(2):83-84.
14. Reiss AL, Abrams MT, Singer HS, Ross JL, Denckla MB. Brain development, gender and IQ in children: a volumetric imaging study. Brain. 1996;119:1763-1774.
15. Wilke M, Sohn JH, Byars AW, Holland SK. Bright spots: correlations of gray matter volume with IQ in a normal pediatric population. Neuroimage. 2003;20(1): 202-215.
16. Martinussen M, Fischl B, Larsson HB, et al. Cerebral cortex thickness in 15-yearold adolescents with low birth weight measured by an automated MRI-based method. Brain. 2005;128(pt 11):2588-2596.
17. Schmithorst VJ, Wilke M, Dardzinski BJ, Holland SK. Cognitive functions correlate with white matter architecture in a normal pediatric population: a diffusion tensor MRI study. Hum Brain Mapp. 2005;26(2):139-147.
18. Reddick WE, White HA, Glass JO, et al. Developmental model relating white matter volume to neurocognitive deficits in pediatric brain tumor survivors. Cancer. 2003;97(10):2512-2519.
19. Peterson BS, Anderson AW, Ehrenkranz R, et al. Regional brain volumes and their later neurodevelopmental correlates in term and preterm infants. Pediatrics. 2003; 111(5, pt 1):939-948.
20. Deary IJ, Bastin ME, Pattie A, et al. White matter integrity and cognition in childhood and old age. Neurology. 2006;66(4):505-512.
21. Maria BL, Neufeld JA, Rosainz LC, et al. Central nervous system structure and function in Sturge-Weber syndrome: evidence of neurologic and radiologic progression. J Child Neurol. 1998;13(12):606-618.
22. Salter MG, Fern R. NMDA receptors are expressed in developing oligodendrocyte processes and mediate injury. Nature. 2005;438(7071):1167-1171.
23. Turner CP, Yan H, Schwartz M, Othman T, Rivkees SA. A1 adenosine receptor activation induces ventriculo-megaly and white matter loss. Neuroreport. 2002; 13(9):1199-1204.
24. Back SA, Craig A, Ling Luo N, et al. Protective effects of caffeine on chronic hypoxiainduced perinatal white matter injury [published online ahead of print October 26, 2006]. Ann Neurol. 2006;60(6):696-705.
25. Wilke M, Krageloh-Mann I, Holland SK. Global and local development of gray and white matter volume in normal children and adolescents [published online ahead of print October 19, 2006]. Exp Brain Res. 2007;178(3):296-307.