Originally Authored by Rajukumar Munian Govindan, Michael E. Behen, Emily Helder, Malek I. Makki, and Harry T. Chugani in Cerebral Cortex
Abstract
Institutional rearing is associated with neurocognitive and behavioral difficulties. Although such difficulties are thought to reflect abnormal neurologic development resulting from early social deprivation (ED) and there is evidence for functional abnormality in children with histories of ED, the impact of early deprivation on brain anatomy has received little study in humans. The present study utilized an objective and sensitive neuroimaging analysis technique (Tract-Based Spatial Statistics) to evaluate white matter fractional anisotropy (FA) and diffusivity in a group of right-handed children with histories of ED (n 5 17; mean age 5 10.9 1 2.6 years) as compared with age-matched healthy controls (n 5 15; mean age 5 11.7 6 2.8 years). Participants underwent magnetic resonance imaging diffusion tensor imaging sequences and comprehensive neuropsychological evaluations. Results revealed reduced FA in frontal, temporal, and parietal white matter including components of uncinate and superior longitudinal fasciculi, in children with histories of ED, providing further support for limbic and paralimbic abnormalities in children with such histories. Furthermore, white matter abnormalities were associated with duration of time in the orphanage and with inattention and hyperactivity scores. It is suspected that the observed white matter abnormalities are associated with multiple depriving factors (e.g., poor prenatal care, postnatal stress) associated with institutional caregiving.
Keywords: Deprivation; DTI; Orphans; TBSS; Uncinate
Introduction
Studies in both animals and humans have demonstrated that early social deprivation (ED) is associated with developmental impairments, neurocognitive dysfunction, and behavioral abnormalities (Ames 1997; Kreppner et al. 2001; Rutter et al. 2001; Behen et al. 2008). Although it is assumed that such findings are reflective of abnormal neurologic development resulting from early social deprivation, the brain regions most affected are poorly understood. Animal studies, including those in nonhuman primates, strongly indicate that early postnatal neglect and deprivation may be associated with both shortterm and long-term changes in brain function and structure (Hofer 1996; Suomi 1997; Kaufman et al. 2000; Chatterjee et al. 2007). Over the last decade, there have been a number of studies evaluating functional neurological outcome in children raised in orphanages. Several recent studies by the Bucharest Early Intervention Project group have demonstrated functional abnormality in children with histories of deprivation as compared with controls. Using electro-encephalography (EEG), Marshall and Fox (2004) demonstrated decreased high frequency power at frontal and temporal lobe sites, and increased low frequency power in posterior sites in children living in institutions versus noninstitution raised children. A later study showed that age at placement into foster care from institution predicted EEG coherence (alpha power and short distance coherence) (Marshall et al. 2008). Additional studies by this group examining event-related potentials (ERPs) in response to facial recognition (Parker and Nelson 2005) and affect discrimination (Parker and Nelson 2005) tasks revealed atypical ERP patterns in children with histories of institutionalization as compared with controls. Such findings, the authors suggest, are consistent with a ‘‘general cortical hypoactivation’’ associated with early institutional rearing. A study using positron emission tomography (PET) scans of children with histories of ED from Romanian orphanages, and subsequently adopted in the United States of America, reported decreased glucose metabolism in a number of brain regions belonging to or strongly associated with limbic and paralimbic systems (Chugani et al. 2001). These brain regions are highly interconnected and have been postulated to be involved in socioemotional functions (e.g., LeDoux 2000).
Thus, although there have been a number of studies indicating functional neurological abnormality, there have been few studies of brain anatomy, perhaps due to the lack of suitable noninvasive methodologies for such investigation. Those that have been done suggest that early deprivation is not only associated with functional brain abnormalities but may also involve structural changes. For instance, a recent study by Tottenham et al. (2009) reported increased amygdala volumes in children having spent time in orphanages, with later adoption associated with increased volumes. Another preliminary study on a small sample of children (n = 7) studied by the Chugani et al. (2001) group, used magnetic resonance (MR) diffusion tensor imaging (DTI) and found decreased fractional anisotropy (FA) in the left uncinate fasciculus (UNF), a limbic pathway connecting medial temporal structures with orbital frontal gyrus, in children who had experienced ED as compared with controls (Eluvathingal et al. 2006), suggesting that structural anatomical abnormalities may extend to white matter. However, this study involved a very small sample and looked only at ‘‘a priori’’ designated tracts of the brain, that is, the tractography analysis was based on manual seed region drawing and thus can be affected to some extent by investigator subjectivity. Furthermore, a comprehensive evaluation of other tracts potentially affected by deprivation was not provided.
The DTI technique provides a sensitive and noninvasive measure of diffusion characteristics of cerebral white matter and is a useful tool for assessment of the structural integrity of white matter. Given the individual differences in brain size and shape, objective and unbiased analysis methods are preferable to manual methods. Recently, voxel-based spatially normalizing techniques such as voxel-based morphometry of statistical parametric mapping (SPM) have been developed and used extensively to study volumetric and topographical differences of cerebral gray matter between subject groups. However, given the heterogeneity of white matter pathways, methods that utilize smoothing lose the structural architecture necessary for the accurate study of cerebral white matter and are not suitable for comparing white matter tracts. To overcome this problem, TBSS is a specialized technique for the study of cerebral white matter by making use of the intrinsic anisotropic property of white matter and projecting the FA values of the tracts onto a virtual skeleton that runs at the median part of the tract (Smith et al. 2006). This technique greatly reduces the total number of voxel comparisons and avoids the spatial smoothing used in other voxel-based techniques. There have been a number of published studies in recent years that have used TBSS to evaluate white matter integrity in wide variety of neurological and psychiatric conditions such as Alzheimer’s disease, multiple sclerosis, substance abuse, bipolar disorder, and parental verbal abuse (Versace et al. 2008; Choi et al. 2009; Roosendaal et al. 2009; Stricker et al. 2009). A recent study in patients with temporal lobe epilepsy using TBSS has shown this method to be more sensitive than SPM in detecting diffusion abnormalities in cerebral white matter (Focke et al. 2008). In the present study, we applied TBSS to study white matter changes in children with histories of early deprivation as compared with normal controls.
Materials and methods
Subjects
ED Group. Seventeen right-handed children (mean age: 10.9 ± 2.6 years, age range: 7.5–15.9, 11 girls) who were raised in Eastern European (n = 10) or Central Asian/Russian (n = 7) orphanages and subsequently adopted in the United States of America underwent comprehensive neuropsychological evaluations and DTI-MR scanning. Participants were recruited through advertisement presented to local and national parent-support groups as well as newsletters for various international adoption groups. All children had spent no time with their biological mothers and were placed in the orphanage immediately subsequent to their hospital stay. Mean duration of time spent in the orphanage was 32.2, standard deviation (SD) = 20.4 months (range: 4–81 months); mean duration of time spent in the adoptive home was 92.0 ± 46.4 months (range: 9–177 months). Mean t-score for head circumference was 29.3, SD = 19.8 at the time of study (Farkas 1994), placing the group mean at approximately the first percentile compared with same age peers. All of the children were English speaking and attended school regularly. Adopted mother’s mean education was 16.1 years, SD = 2.3, and adopted father’s education was 16.3 years, SD = 1.9. Nine of the 17 children were taking psychoactive medication. Table 1 presents the demographics and neurocognitive characteristics of the overall ED group. As can be seen, as a group, the children were functioning in the average range in most domains measured. Exceptions included Freedom from Distractibility Index, verbal memory, receptive language processing, and sustained attention and arithmetic, all of which were measured in the low average range. Impulsivity was measured in the mildly impaired range. Parent report of behavioral problems revealed that hyperactivity, attention problems, and conduct problems were all measured in the ‘‘at-risk’’ range for the group. Anxiety and depression subscales were both measured within normal limits. In order to minimize the occurrence of major confounding factors with known neurobiological effects, children with current and/or historical indication of any of the following were excluded from the study: prematurity, pre or perinatal difficulties, spent time with the biological mother prior to adoption (according to adoptive parent report and medical records), current or historical medical problems, epilepsy, documented suspicion of or known prenatal exposure to alcohol and/or other substances, and/or focal findings on neurologic examination. Alcohol exposure was directly assessed by a neurologist as part of the neurological examination using the Miller criteria (Miller et al. 2006). Children with a ‘‘high’’ phenotypic suggestion of alcohol exposure ( >12) were not included in the study. Despite the efforts made to exclude children based on a number of the above potential confounds (e.g., low birth weight, knowledge of prematurity, and/or identified suspicion of alcohol exposure prenatally), as with most studies of institutionally adopted children, historical records were poor and it is likely that we had not completely excluded these confounds from the sample.
Control Group. Fifteen right-handed typically developing nonadopted children (mean age = 11.7 ± 2.8 years; SD, age range: 6–15.9 years, 7 girls) all born in the United States of America were recruited to serve as controls. All of the children underwent neuropsychological evaluations, as well as a semistructured interview to ensure that they all had normal intellectual function, and to rule out any current and/or historical developmental, medical, or psychiatric conditions. Inclusion criteria for this group were measured global intellectual functioning (Full scale intelligence quotient, FSIQ>85) within normal limits; English speaking; right handed; regular school attendance; absence of current or historical medical and/or psychiatric diagnoses; absence of current or historical use of psychoactive medications. FSIQ for the full group was 109.66 ± 11.73. Parent report of behavioral problems resulted in t-scores for internalizing (t-score = 46.1 ± 6.82) and externalizing (t-score = 45 ± 7.42) behaviors were well within the normative range. All of the children in the control group received compensation ($100.00) for their participation in the study.
Procedure
Participants were initially screened by telephone for inclusion– exclusion criteria. Those participants that were likely to meet all inclusion and exclusion criteria were then scheduled for neuropsychological evaluations and neurological examinations. Written informed consent was obtained from all participants prior to enrollment in the study. Thirty-five total participants (18 ED, 17 controls) were initially included in the current study and met all inclusion and exclusion criteria for participation. Two control subjects and one ED subject were excluded due to motion artifacts on magnetic resonance imaging (MRI, see below). The study involved 4 components: 1) a neuropsychological evaluation and neurologic screening, 2) functional MRI (fMRI), 3) T1 weighted Magnetization Preparation Rapid Acquisition Gradient Echo (MP-RAGE) scan, and 4) DTI sequence (see below). Participation involved 2 visits to the Children’s Hospital of Michigan. On the first visit, all the children enrolled in the study first underwent a neuropsychological evaluation and neurologic screen to ensure that they met all the criteria for further participation in the study. The first visit took 4–6 h to complete. The second visit consisted of fMRI, MP-RAGE, and DTI scans. None of the participants were sedated for any of the procedures in this study. This visit took approximately 45–60 min. Results of volumetric MRI and fMRI sequences are discussed elsewhere.
Neuropsychological Evaluation. The neuropsychological evaluation for the orphan group consisted of evaluation of global, verbal, and nonverbal intellectual functioning, expressive and receptive vocabulary and language processing, verbal and verbal memory, achievement, executive functioning (attention, impulsivity), manual dexterity, and behavioral functioning. The battery included the following measures: Weschler Intelligence Scales for Children—Third Edition (WISC-III); Token Test for Children, Comprehensive Evaluation of Language Functions—Third Edition (CELF-3), Wide Range Assessment of Learning and Memory (WRAML), Wide Range Achievement Test—Third Edition, Gordon Diagnostic System (GDS), Grooved Pegboard. In addition, the Behavioral Assessment Scales for Children (BASC) was administered to evaluate internalizing and externalizing behavioral problems. The psychometric properties of the above measures have been well established and the above measures are widely used with both clinical and research populations (Sattler 2001). The evaluation also included a social–historical interview with the child’s adoptive parent(s). Children’s head circumference (early deprivation group) and height and weight (for body mass index, BMI, for both groups), and current/historical medication use were measured by the evaluating neurologist at the time of first interview. Summary scores for the following were used to quantify neurocognitive functioning in 9 domains: ‘‘Global intellectual functioning’’ (WISC-III, FSIQ), ‘‘Receptive Language’’ (Token Test, Total score), ‘‘Expressive Language’’ (CELF-3, composite of Producing Word Associations and Rapid Automatic Naming), ‘‘Verbal Memory’’ (WRAML, Verbal Memory Index), ‘‘Visual Memory’’ (WRAML, Visual Memory Index), ‘‘Sustained Attention’’ (GDS, Vigilance Hits), ‘‘Impulsivity’’ (GDS, Vigilance false alarms), Manual dexterity (Grooved Pegs, ‘‘dominant’’ and ‘‘nondominant hand’’). Behavioral problems and executive behaviors were quantified via the following 5 domains: ‘‘Anxiety,’’ ‘‘Depression,’’ ‘‘Attention Problems,’’ ‘‘Hyperactivity,’’ and ‘‘Conduct Problems’’ (BASC).
Imaging protocol. MRI scans were performed using a 1.5-T magnet (Siemens Sonata, Erlangen, Germany) and standard head coil. Diffusion tensor images were acquired with double refocusing spin-echo echo-planar imaging in the axial plane with diffusion sensitization gradients applied in 6 noncollinear directions with a b-value of 1000 s/mm2 . The same imaging parameters were applied to acquire T2 weighted (b ~ 0 s/ mm2 ) images to use as a reference image for signal attenuation measurement. All image volumes were acquired using 8 repetitions to increase the number of measures. Acquiring images in each direction with 6 repetitions improves the image quality (geometric distortion and eddy current artifacts), increases the signal-to-noise ratio and avoids underestimation of anisotropy values. The echo time was 97 ms, and the repetition time was 6.6 s. A set of minimum 34 axial slices of 3- mm thickness without a gap, covering the whole brain including the cerebellum, was acquired with matrix size 128 3 128 and voxel size 1.8 3 1.8 3 3 mm3 . Field of view is 240 3 240 mm2 and the approximate scanning time for the DTI acquisition was 7 min.
Tensor Calculation and TBSS
Following image acquisition, the diffusion images were transferred to a PC workstation and further processed. The entire image sets were visually inspected and affine corrected for motion and other imaging artifacts; participants with uncorrectable images were excluded from the study (n = 2, both control participants). The tensor calculation of the DTI images was performed using DtiStudio software provided by Johns Hopkins University (Jiang et al. 2006). The native B0 image, and the images derived from the tensor calculation, the FA image, and the apparent diffusion coefficient (ADC) image were saved and transferred to a Linux-based workstation for further processing. The next step involved extraction of the brain matter on the B0 image, using the Brain Extraction Tool available with the FSL software; a fractional intensity threshold of 0.35 was used for this step. Using the brain extracted B0 image, we then extracted the brain for the FA and the ADC images. The brain extracted FA and ADC images of all participants were used as the input images for TBSS processing (Smith et al. 2006).
The initial step of TBSS analysis consisted of voxelwise nonlinear registration of all participants to a target image provided by the FSL software. The transformed FA images of all participants were averaged to create a mean FA image. This mean FA image was then used to create a skeleton image of the white matter tracts using a protocol that searches and labels the skeleton voxels with maximum FA intensity along the perpendicular direction (breath) of a white matter tract. An FA threshold of 0.2 was used to differentiate between gray and white matter. The mean skeleton, derived from the mean FA image, is identified at the exact median of the white matter tract. This mean skeleton was later applied to the registered FA image of individual participants. Following the search for the maximum FA value along the width of the white matter tract, perpendicular to the skeleton voxel, the maximum FA values were then projected onto the skeleton voxel for further statistical analysis. In a separate process using the FA image derived skeleton, the maximum values along the direction perpendicular to the tract of the ADC image was projected to a separate skeleton image. Voxelwise statistical analysis of individual skeleton images of both controls and ED children for both contrasts (controls > ED) and (controls < ED), with age and medication use as covariates, was performed using a nonparametric permutation test with a cluster size threshold of >3 and P value of <0.05 for significance, after correcting for multiple comparisons. Skeletal voxels that were significantly different between the groups were isolated and labeled; these voxels were then expanded to include the full width of the white matter tract, which was then used as a mask permitting calculation of the mean FA and ADC values for the width of the tract for individual participants. These regional/tract values were then correlated with demographic characteristics (age, head circumference, BMI, medication use, duration of time in the orphanage, and duration of time in the adoptive home) and neuropsychological domains across both groups; those that were correlated with any of the DTI metrics were included as covariates in subsequent correlational analyses. In order to apply some correction for Type I error associated with multiple comparisons, pairwise alpha for correlations between demographic and behavioral variables and DTI metrics was P = 0.01. Statistical analysis was performed using SPSS version 15.
Results
TBSS Analysis
The TBSS analysis showed multiple regions of decreased FA in white matter tracts of the ED group as compared with controls (Fig. 1, Table 2). These regions involved bilateral UNF (P < 0.05) (Fig. 1a–c) with extensive involvement of the right UNF, including the white matter region adjacent to the right amygdala (see Fig. 1a, and Table 2). In addition, decreased FA was also seen in regions involving bilateral superior longitudinal fasciculus (SLF, P < 0.05) (Fig. 1d–h). Results showed no significant regions with increased FA value compared with the controls and analysis of ADC images showed no significant regional differences compared with the controls.
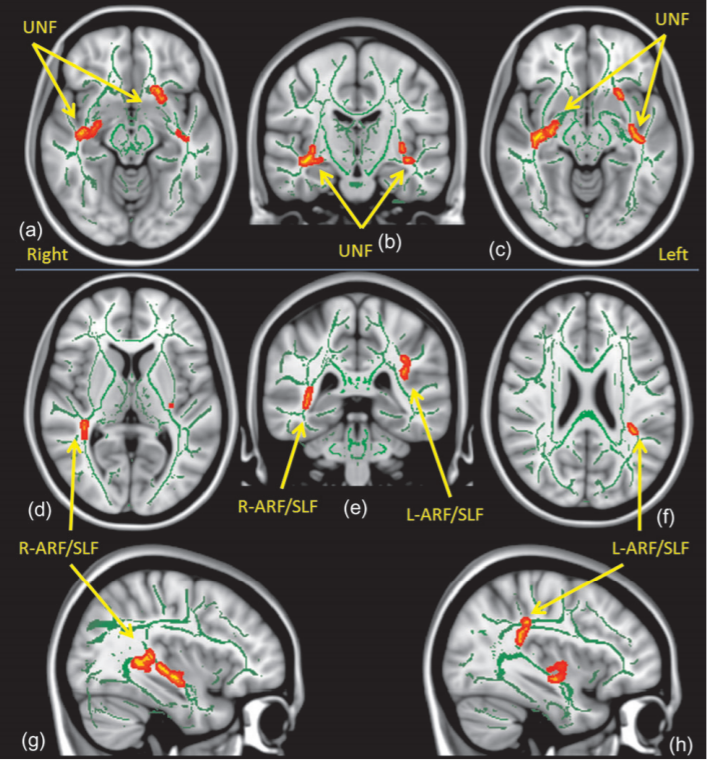
fasciculus (a–c), ARF: arcuate fasciculus, and SLF: superior longitudinal fasciculus (d–h).
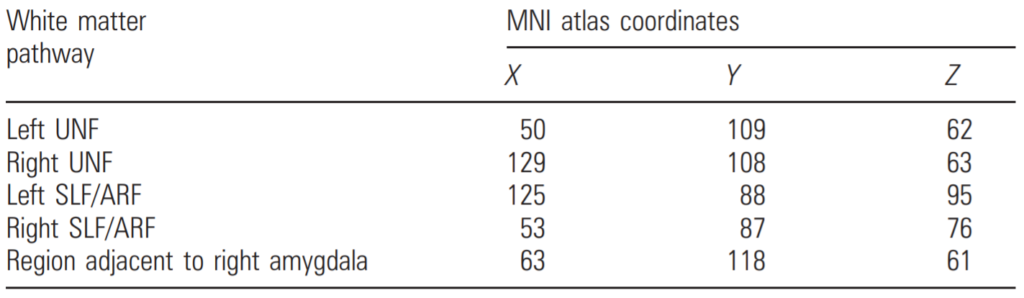
Note: MNI atlas coordinates for regions with decreased FA values (TBSS, cluster threshold [ 3,
P value \ 0.05, after correcting for multiple comparison and with age as a covariate) in 17 ED
Children compared with 15 control subjects.
Correlation with Demographics Variables
Correlations between right and left UNF and right and left SLF with age, gender, region of adoption, and head circumference did not reach significance. FA for the left and right UNF (P = 0.0001, P = 0.005) and left SLF (P = 0.01) were significantly positively correlated with BMI. FA for the right UNF also showed a significant negative correlation with duration of stay in the orphanage (r = –0.604, P = 0.01) (see Fig. 2). There was also a trend for increased FA in right UNF (P = 0.045) in children not using medication versus those who were currently or had taken psychoactive medication.
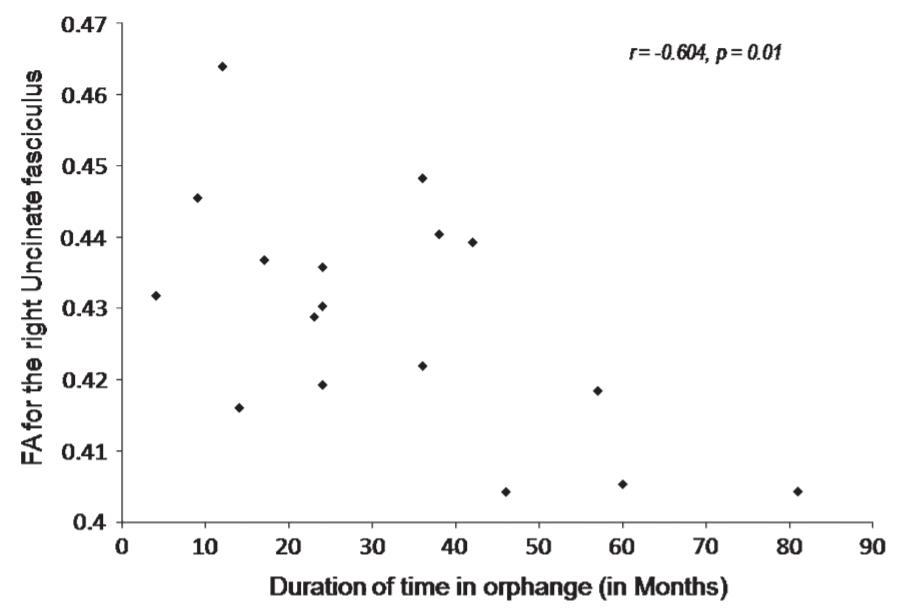
fasciculus with duration of stay in the orphanage (r = -0.604, P 5 0.01).
TBSS Analysis with BMI and Medication use as Covariates
Given the correlation between BMI and FA for UNF and SLF, and between medication use and FA for these regions, the TBSS analysis was rerun with BMI and medication use included as covariates in separate analyses. With medication included as a covariate, the bilateral decrease in UNF in the ED group remained significant (P < 0.05); however, for the SLF, only the left was significantly different between the groups (P < 0.05); although there remained a trend for decreased right SLF (P < 0.10) fir the ED group as compared with the controls. With BMI included as a covariate, only the bilateral UNF remained significant at the P < 0.05 level; although the trend for bilaterally decreased SLF in the ED group remained (P < 0.10).
Correlations with Neuropsychological Variables
Correlations between FA for the left and right UNF and SLF and neuropsychological domains revealed significant negative correlations between hyperactivity and attention with left UNF (r = –0.623, P = 0.0001—see Fig. 3; r = –0.639, P = 0.0001), hyperactivity and attention with right UNF (r = –0.504, P = 0.004; r = –0.499, P = 0.004), hyperactivity with right and left SLF (r = –0.486, P = 0.006; r = –0.538, P = 0.002), attention with right SLF (r = –0.469, P = 0.008) and with trends for increased problems in attention and left SLF (r = –0.402, P = 0.025) as well.
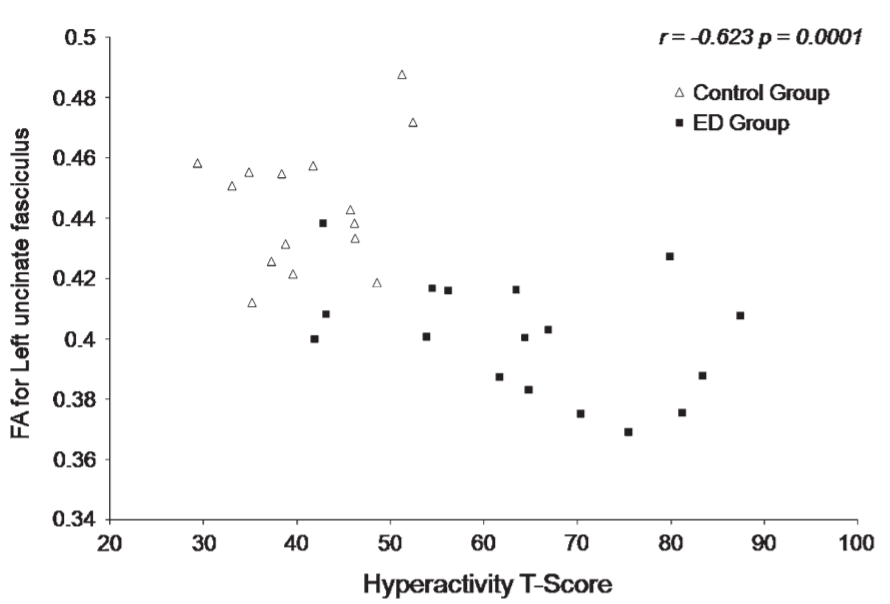
with decreased FA value in the left UNF of the ED children compared with control
children (r = -0.623, P 5 0.0001).
Discussion
In the present study, we found multiple structural abnormalities in white matter comprising portions of the frontal, temporal, and parietal cortices. These findings are consistent with and extend the observations from previous studies, using volumetric MRI (Tottenham et al. 2009), fluorodeoxyglucose PET (Chugani et al. 2001), ERPs (Marshall and Fox 2004; Marshall et al. 2008), and MR-DTI tractography (Eluvathingal et al. 2006). Moreover, we found that the ‘‘magnitude’’ of white matter disorganization in these regions was significantly correlated with duration of stay in the orphanage, as well as other neuropsychological outcomes previously reported to be commonly present in children with such histories (O’Connor and Rutter 2000; Behen et al. 2008)
Although, the precise neurobiological significance of decreased FA is still not well understood, FA is thought to represent an indirect measurement of white matter structural integrity (Beaulieu 2002) and there are many studies showing associations of FA with age-related maturation (Schneider et al. 2004; Eluvathingal et al. 2007) and various pathological conditions (reviewed in Alexander et al. 2007). The proposed mechanisms attributed for decreased FA are loss or lack of myelination (Jito et al. 2008), loss of axons, gliosis, and tissue edema. In addition, nascent axon sprouting, axonal spin formation, and architectural variations, such as increased axonal crossing, can lead to decreased FA (Madler et al. 2008). In the present study, the major findings were only seen in FA values; the groups did not differ on ADC. This pattern argues against inflammation or vascular edema as the mechanism for the abnormal findings in these regions (Yu and Tan 2008). Diffusion changes seen in the present study may be due to one or more components of the multifaceted deprivation associated with the orphanage experience including lack of exposure to experience-expected stimulation (Greenough et al. 1987). Inadequate exposure to experience-expected visual stimuli has been shown to reduce FA in the optic radiation in humans with early blindness (Shimony et al. 2006; Park et al. 2007; Shu et al. 2009), and numerous other studies have shown that deprivation of sensory, motor, and social stimulation has profound effects on both function and structure of the developing nervous system and in domainspecific systems (Nakadate et al. 2001; Vandermeeren et al. 2003; Lee et al. 2005; Sharma et al. 2005).
Alternatively, the findings could be secondary to adverse and chronic experience of stress. Dysregulation of stress response as a result of social deprivation has been demonstrated in postinstitutionalized children (Carlson and Earls 1997; Gunnar et al. 2001). Further, prolonged stress is known to have adverse (typically focal) consequences for the developing central nervous system (Bremner and Vermetten 2004). A recent study found a negative correlation between exposure to stressful life events during childhood and FA for the genu of the corpus callosum (Paul et al. 2008). Important to note is that the abnormalities found in the present and previous work (see Marshall and Fox 2004; Eluvathingal et al. 2006; Tottenham et al. 2009) involve those regions that appear to be particularly susceptible to the physiological effects of stress (Kaufman and Charney 2000; McEwen 2000).
Methodological Limitations
Although the automated methodology used for image processing in the present study has several advantages over other methods, it is still relatively new and warrants additional comment. For example, this procedure conceptually assumes that in a given white matter tract the anisotropic value is at the maximum in the center of the tract and gradually decreases away from the tract center. However, this assumption is not always true and in several regions where 2 or more tracts converge or diverge, a more sophisticated FA projection technique is needed (Smith et al. 2006). In addition, the morphology of the derived skeleton might also change in the tract adjacent to the ventricle (e.g., fornix or posterior aspect of cingulum), due to the partial volume effect of cerebrospinal fluid or due to severe structural changes that might be caused by the primary pathological condition. Thus, in this study, although we report multiple regions of FA abnormality, there may be other abnormal regions not identified by this method. It is less likely that this methodological complexity has resulted in spurious positive findings. Additionally, in regions of the brain where multiple tracts converge, given the low spatial resolution inherent to DTI, it is not possible to definitively discriminate fibers of one tract from another. For instance, the finding of reduced FA in the area of the SLF may actually represent the arcuate fasciculus or other component(s) of the larger SLF (SLF I, II, or III) (Schmahmann et al. 2007). Similarly, although the abnormality identified adjacent to amygdala likely represents UNF (Schmahmann, 2006), involvement of other efferent-afferent pathways of the amygdala cannot be ruled out. Given these problems, a tractography analysis method that evaluates the whole tract may yield more specific results. Such work involving other tractographic modalities (deterministic tracking, based on reconstruction of fiber pathway based on primary diffusion direction using operator-dependent region of interest, Mori et al. 1999) of these pathways is currently underway in our laboratory. Though given the disadvantages of the deterministic approach (i.e., more vulnerable to observer bias due to variable seed selection), a strategy employing both methods could capitalize on the advantages of each.
The groups differed with regard to current/historical medication use, which was marginally related to DTI metrics; however, the findings remained significantly different and largely unchanged when controlling for medication use. Finally, although rigorous quality control was used for motion correction and other imaging artifacts, it remains possible that our results are confounded to some degree by movement artifact. In spite of these limitations, however, the TBSS analysis identified regions in the ED group that were significantly different from controls, and which replicate work from previous studies using disparate methods (e.g., Chugani et al. 2001; Eluvathingal et al. 2006); such findings increase confidence in the present observations.
The present community-recruited sample of ED children was measured in the average range across most neuropsychological domains. Behaviorally, hyperactivity and attention problems were measured for the overall group in the at-risk range. This level of overall global cognitive functioning and behavioral problems is comparable with that presented in other selected or random samples of children with histories of early deprivation (Ames 1997; Rutter et al. 2001; Beckett et al. 2006; Gunnar and van Dulmen 2007; Colvert et al. 2008). Thus, although not randomly selected, the present sample does not appear to be biased in any pathological direction, either cognitively or behaviorally.
The children in the present study were raised in orphanages in several regions. There is some support for differential outcome in terms of function (e.g., behavior problems) across regions (Gunnar and van Dulmen 2007). Although there were no significant differences between groups defined by region of adoption on the DTI variables, given the small sample sizes for this comparison, it cannot be ruled out that region of adoption influenced the results of the present study. Further evaluation of this possibility with larger samples is warranted. Also, although none of the children in the study spent any time with their biological mothers, it has not been possible to ascertain, in many cases, the exact length of hospital stay prior to their going to the orphanage. Although in all cases the stay was reported to be less than 2 months, we cannot rule out that some of the children had longer hospital stays prior to going to the orphanage. Finally, the children in the ED group, although having measured neurocognitive functioning well with within the average range in most domains, did present with mildly deficient performance on a measure of impulsivity and therefore present diffusion findings could be associated more with deficient behavioral control than history of deprivation. Addition of a control group with similar neurocognitive functioning (i.e., Attention deficit hyperactive disorder) would permit us to determine if diffusion abnormalities are associated more with history of deprivation or current behavioral difficulties.
Neuropsychological and Structural Abnormalities Associated with ED
Animal studies have documented structural and physiological alterations to the nervous system associated with maternal deprivation. For instance, several studies have demonstrated that early social isolation produces neurophysiological alterations in rodents and monkeys, including alterations in stress responsivity (Hofer 1994; Pryce and Feldon 2003), altered chemoarchitecture of neurotransmitter systems in the striatum (Martin et al. 1991) and medial prefrontal cortex (Braun et al. 2000). Others have reported structural alterations associated with postnatal deprivation. Chatterjee et al. (2007) found elevations in the number of neurons and supporting astroglial cells and reduced indices of functionality in motor cortex, medial prefrontal cortex, medial preoptic area, ventromedial hypothalamus, nucleus accumbens, and amygdala in maternally deprived rat pups. Further, reduced functionality was associated with reduced neuronal pruning and apoptosis. Card et al. (2005) found that handling/maternal separation in rodents was associated with reduced number of preautonomic limbic forebrain neurons. Such findings strongly support experiencedependent changes in the limbic brain secondary to environmental stress/deprivation. Noteworthy is that the above studies point to disruption of the same limbic and paralimbic systems that have been reported to be abnormal in children with histories of early deprivation.
Studies of children raised in orphanages have reported decreased glucose hypometabolism in prefrontal and medial and lateral temporal lobe (including the amygdala) (Chugani et al. 2001), decreased high frequency power at frontal and temporal lobe sites (Marshall and Fox 2004), as well as reduced organization of the left UNF using deterministic tractography (Eluvathingal et al. 2006). A recent study by Tottenham et al. (2009) found increased amygdala volumes in children with histories of institutional rearing, extending structural findings to humans. The findings of the present study provide further evidence that structural abnormalities extend to white matter as well. Abnormal gray matter volumes in limbic structures (e.g., amygdala, Tottenham et al. 2009) could underlie the functional abnormalities (Chugani et al. 2001; Marshall and Fox 2004) as well as the abnormal white matter findings presented here. Further study of the relationship between gray matter (i.e., morphometry, cortical thickness) of these and additional limbic (i.e., orbital frontal and anterior temporal cortices) structures is warranted in order to further investigate the relationship between gray matter abnormalities and white matter organization.
The diffusion abnormalities identified in the present study appear to involve those regions of the brain thought to subserve functions frequently found to be impaired in children with histories of ED. The UNF reciprocally connects the inferior frontal lobe with medial temporal structures lobe (Ebeling and Voncramon 1992; Schmahmann 2006). These brain regions have been shown to be involved in socioemotional, memory, and executive functions (Rolls 2004; Schmahl and Bremner 2006; Makris et al. 2007). The SLF is a broad association pathway that reciprocally connects the parietal, temporal, and occipital cortices to the frontal lobe. In the present study, as noted, the abnormality in the area of the left SLF may involve the arcuate fasciculus (Fig. 1) (Schmahmann et al. 2007; Catani and Mesulam 2008). The arcuate fasciculus connects the caudal superior temporal cortex with the dorsal prefrontal cortex and is known to be involved in language functions (Schmahmann 2006). Language delay and impairment have been reported in a number of studies in children with histories of early deprivation (Ames 1997; Croft et al. 2007; Behen et al. 2008). The association in the present study between hyperactivity and attention problems and FA of fronto-temporal white matter is consistent with the possibility that diffusion abnormalities and associated dysfunction in the affected brain regions may underlie the cognitive, socioemotional, and behavioral difficulties that have been commonly observed in children with early severe socioemotional deprivation. Although there has as yet been little research that has examined the functional brain correlates of these findings (e.g., with fMRI), this work is currently underway in our laboratory.
Association of Duration of Deprivation with Structural Findings
The finding that FA for the UNF was significantly negatively correlated with duration of stay in the orphanage is consistent with previous work that has shown dose–effect relationships between time in the orphanage and neurocognitive and behavioral outcomes (O’Connor and Rutter 2000; Behen et al. 2008), and with the recent study by (Tottenham et al. 2009) that showed an association between age at adoption and amygdala volumes. Results of the present study extend the relationship between length of deprivation and outcome to white matter organization.
Multi-factorial Deprivation and Structural Neural Abnormality
As noted in the Materials and Methods section, despite attempts to exclude children with histories of low birth weight, prematurity, and/or alcohol exposure it is likely that we had not completely excluded these confounds from the sample. Also, in addition to pre and perinatal factors, some degree of malnutrition is known to have occurred in a majority (if not all) of the participants as is evidenced by small physical stature and head circumference. The findings of the present study may be associated with such factors. Poor prenatal nutrition has been linked to poor myelination and neurotransmission (Lozoff 2007). Additionally, substantial and impressive data have supported links between maternal stress during pregnancy and altered stress responsivity and limbic structural abnormality (Van den Bergh et al. 2005). Exposure to alcohol has also been shown to be associated with reductions in gray and white matter including the frontal and temporal lobe (see Spadoni et al. 2007). Postnatal malnutrition has been shown to be associated with structural brain changes, including frontal and temporal cortices (Kirksey et al. 1990; Cordero et al. 1993; Granados-Rojas et al. 2004). Although the findings in the present study were not associated with head circumference, DTI findings were associated with BMI suggesting that indeed malnutrition likely played a role in the observed structural findings. However, statistical control for BMI did not substantially alter the findings, suggesting that malnutrition does not fully account for the findings. In any case, given the research that has shown effects of malnutrition on the developing nervous system (Guerrini et al. 2007), further research on larger samples is warranted to further evaluate potential associations between malnutrition and neural abnormalities in limbic and paralimbic structures/tracts in children with histories of early deprivation.
Ames (1997) compared children adopted from orphanages with children adopted internationally at birth directly from the hospital, and found that the latter group closely resembled normal controls across most cognitive and behavioral measures suggesting that orphanage experience is more important to outcome (in terms of cognitive and socioemotional function) than prenatal factors. Additionally, studies with the English and Romanian adoptee sample have showed that weight at adoption, an index of subnutrition, had no relationship to cognitive functioning at age 11 (Beckett et al. 2006), and further that reduced head circumference can occur in the absence of subnutrition (Sonuga-Barke et al. 2008). Such findings provide some support for a role of psychosocial factors in addition to the above biological ones in producing negative outcomes. Further research on larger samples is warranted to determine if such a relationship extends beyond functional (e.g., neuropsychological) to structural (e.g., fiber tracts) outcomes.
Conclusion
The present study utilized tract-based spatial statistics to evaluate white matter integrity in a group of children with histories of early severe deprivation as compared with healthy controls. Results revealed reduced organization of frontal, temporal, and parietal white matter including components of UNF and SLF, in children with histories of early deprivation, providing further support for limbic and paralimbic abnormalities in children with such histories. DTI metrics were associated with duration of time spent in the orphanage and inattention and hyperactivity. It is suspected that multiple depriving factors associated with institutional caregiving contribute to both functional and structural findings in children with histories of early deprivation. Future work will focus on functional correlates (e.g., fMRI) of structural findings.
Fundings
National Institute of Mental Health (Grant Number K08MH079176 to M.E.B.).
Notes
The content is solely the responsibility of the authors and does not
necessarily represent the official views of the National Institute of
Mental Health or the National Institutes of Health. Conflict of Interest:
None declared. Address correspondence to Michael E. Behen, PhD. Pediatrics and Neurology, School of Medicine, Wayne State University, Children’s Hospital of Michigan. Email: mbehen@pet.wayne.edu.
References
Alexander AL, Lee JE, Lazar M, Field AS. 2007. Diffusion tensor imaging of the brain. Neurotherapeutics. 4:316–329.
Ames EW. 1997. The development of Romanian orphanage children adopted into Canada. Final report to National Welfare Grants Program: Human Resources Development Canada. Barnaby, British Columbia: Simon Fraser University.
Beaulieu C. 2002. The basis of anisotropic water diffusion in the nervous system—a technical review. NMR Biomed. 15:435–455.
Beckett C, Maughan B, Rutter M, Castle J, Colvert E, Groothues C, Kreppner J, Stevens S, O’Connor TG, Sonuga-Barke EJ. 2006. Do the effects of early severe deprivation on cognition persist into early adolescence? Findings from the English and Romanian adoptees study. Child Dev. 77:696–711.
Behen ME, Helder E, Rothermel R, Solomon K, Chugani HT. 2008. Incidence of specific absolute neurocognitive impairment in globally intact children with histories of early severe deprivation. Child Neuropsychol. 14:453–469.
Braun K, Lange E, Metzger M, Poeggel G. 2000. Maternal separation followed by early social deprivation affects the development of monoaminergic fiber systems in the medial prefrontal cortex of Octodon degus. Neuroscience. 95:309–318.
Bremner JD, Vermetten E. 2004. Neuroanatomical changes associated with pharmacotherapy in posttraumatic stress disorder. Ann NY Acad Sci. 1032:154–157.
Card JP, Levitt P, Gluhovsky M, Rinaman L. 2005. Early experience modifies the postnatal assembly of autonomic emotional motor circuits in rats. J Neurosci. 25:9102–9111.
Carlson M, Earls F. 1997. Psychological and neuroendocrinological sequelae of early social deprivation in institutionalized children in Romania. Ann NY Acad Sci. 807:419–428.
Catani M, Mesulam M. 2008. The arcuate fasciculus and the disconnection theme in language and aphasia: history and current state. Cortex. 44:953–961.
Chatterjee D, Chatterjee-Chakraborty M, Rees S, Cauchi J, de Medeiros CB, Fleming AS. 2007. Maternal isolation alters the expression of neural proteins during development: ‘stroking’ stimulation reverses these effects. Brain Res. 1158:11–27.
Choi J, Jeong B, Rohan ML, Polcari AM, Teicher MH. 2009. Preliminary evidence for white matter tract abnormalities in young adults exposed to parental verbal abuse. Biol Psychiatry. 65:227–234.
Chugani HT, Behen ME, Muzik O, Juhasz C, Nagy F, Chugani DC. 2001. Local brain functional activity following early deprivation: a study of postinstitutionalized Romanian orphans. NeuroImage. 14: 1290–1301.
Colvert E, Rutter M, Kreppner J, Beckett C, Castle J, Groothues C, Hawkins A, Stevens S, Sonuga-Barke EJ. 2008. Do theory of mind and executive function deficits underlie the adverse outcomes associated with profound early deprivation?: findings from the English and Romanian adoptees study. J Abnorm Child Psychol. 36:1057–1068.
Cordero ME, D’Acuna E, Benveniste S, Prado R, Nunez JA, Colombo M. 1993. Dendritic development in neocortex of infants with early postnatal life undernutrition. Pediatr Neurol. 9:457–464.
Croft C, Beckett C, Rutter M, Castle J, Colvert E, Groothues C, Hawkins A, Kreppner J, Stevens SE, Sonuga-Barke EJ. 2007. Early adolescent outcomes of institutionally-deprived and non-deprived adoptees. II: language as a protective factor and a vulnerable outcome. J Child Psychol Psychiatry. 48:31–44.
Ebeling U, Voncramon D. 1992. Topography of the uncinate fascicle and adjacent temporal fiber tracts. Acta Neurochirurgica. 115: 143–148.
Eluvathingal TJ, Chugani HT, Behen ME, Juhasz C, Muzik O, Maqbool M, Chugani DC, Makki M. 2006. Abnormal brain connectivity in children after early severe socioemotional deprivation: a diffusion tensor imaging study. Pediatrics. 117:2093–2100.
Eluvathingal TJ, Hasan KM, Kramer L, Fletcher JM, Ewing-Cobbs L. 2007. Quantitative diffusion tensor tractography of association and projection fibers in normally developing children and adolescents. Cereb Cortex. 17:2760–2768.
Farkas L. 1994. Anthropometry of the head and face. New York: Raven press.
Focke NK, Yogarajah M, Bonelli SB, Bartlett PA, Symms MR, Duncan JS. 2008. Voxel-based diffusion tensor imaging in patients with mesial temporal lobe epilepsy and hippocampal sclerosis. NeuroImage. 40:728–737.
Granados-Rojas L, Aguilar A, Diaz-Cintra S. 2004. The mossy fiber system of the hippocampal formation is decreased by chronic and postnatal but not by prenatal protein malnutrition in rats. Nutr Neurosci. 7:301–308.
Greenough WT, Black JE, Wallace CS. 1987. Experience and brain development. Child Dev. 58:539–559.
Guerrini I, Thomson AD, Gurling HD. 2007. The importance of alcohol misuse, malnutrition and genetic susceptibility on brain growth and plasticity. Neurosci Biobehav Rev. 31:212–220.
Gunnar MR, Morison SJ, Chisholm K, Schuder M. 2001. Salivary cortisol levels in children adopted from romanian orphanages. Dev Psychopathol. 13:611–628.
Gunnar MR, van Dulmen MH. 2007. Behavior problems in postinstitutionalized internationally adopted children. Dev Psychopathol. 19:129–148.
Hofer MA. 1994. Early relationships as regulators of infant physiology and behavior. Acta Paediatr Suppl. 397:9–18.
Hofer MA. 1996. On the nature and consequences of early loss. Psychosom Med. 58:570–581.
Jiang H, van Zijl PC, Kim J, Pearlson GD, Mori S. 2006. DtiStudio: resource program for diffusion tensor computation and fiber bundle tracking. Comput Methods Prog Biomed. 81:106–116.
Jito J, Nakasu S, Ito R, Fukami T, Morikawa S, Inubushi T. 2008. Maturational changes in diffusion anisotropy in the rat corpus callosum: comparison with quantitative histological evaluation. J Magn Res Imag. 28:847–854.
Kaufman J, Charney D. 2000. Comorbidity of mood and anxiety disorders. Depress Anxiety. 12(Suppl 1):69–76.
Kaufman J, Plotsky PM, Nemeroff CB, Charney DS. 2000. Effects of early adverse experiences on brain structure and function: clinical implications. Biol Psychiatry. 48:778–790.
Kirksey A, Morre DM, Wasynczuk AZ. 1990. Neuronal development in vitamin B6 deficiency. Ann NY Acad Sci. 585:202–218.
Kreppner JM, O’Connor TG, Rutter M. 2001. Can inattention/overactivity be an institutional deprivation syndrome? J Abnorm Child Psychol. 29:513–528.
LeDoux JE. 2000. Emotion circuits in the brain. Annu Rev Neurosci. 23:155–184.
Lee HJ, Kang E, Oh SH, Kang H, Lee DS, Lee MC, Kim CS. 2005. Preoperative differences of cerebral metabolism relate to the outcome of cochlear implants in congenitally deaf children. Hear Res. 203:2–9.
Lozoff B. 2007. Iron deficiency and child development. Food Nutr Bull. 28:S560–S571.
Madler B, Drabycz SA, Kolind SH, Whittall KP, MacKay AL. 2008. Is diffusion anisotropy an accurate monitor of myelination? Correlation of multicomponent T-2 relaxation and diffusion tensor anisotropy in human brain. Magn Reson Imaging. 26(7):874–888.
Makris N, Papadimitriou GM, van der Kouwe A, Kennedy DN, Hodge SM, Dale AM, Benner T, Wald LL, Wu O, Tuch DS, et al. 2007. Frontal connections and cognitive changes in normal aging
rhesus monkeys: a DTI study. Neurobiol Aging. 28:1556–1567. Marshall PJ, Fox NA. 2004. A comparison of the electroencephalogram between institutionalized and community children in Romania. J Cogn Neurosci. 16:1327–1338.
Marshall PJ, Reeb BC, Fox NA, Nelson CA, 3rd, Zeanah CH. 2008. Effects of early intervention on EEG power and coherence in previously institutionalized children in Romania. Dev Psychopathol. 20: 861–880.
Martin LJ, Spicer DM, Lewis MH, Gluck JP, Cork LC. 1991. Social deprivation of infant rhesus monkeys alters the chemoarchitecture of the brain: I. Subcortical regions. J Neurosci. 11:3344–3358.
McEwen BS. 2000. Effects of adverse experiences for brain structure and function. Biol Psychiatry. 48:721–731.
Miller LC, Chan W, Litvinova A, Rubin A, Comfort K, Tirella L, Cermak S, Morse B, Kovalev I. 2006. Fetal alcohol spectrum disorders in children residing in Russian orphanages: a phenotypic survey. Alcohol Clin Exp Res. 30:531–538.
Mori S, Crain BJ, Chacko VP, van Zijl PC. 1999. Three-dimensional tracking of axonal projections in the brain by magnetic resonance imaging. Ann Neurol. 45:265–269.
Nakadate K, Imamura K, Watanabe Y. 2001. Effects of monocular deprivation on the expression pattern of alpha-1 and beta-1 adrenergic receptors in the kitten visual cortex. Neurosci Res. 40: 155–162.
O’Connor TG, Rutter M. 2000. Attachment disorder behavior following early severe deprivation: extension and longitudinal follow-up. English and Romanian Adoptees Study Team. J Am Acad Child Adolesc Psychiatry. 39:703–712.
Park HJ, Jeong SO, Kim EY, Kim JI, Park H, Oh MK, Kim DJ, Kim SY, Lee SC, Lee JD. 2007. Reorganization of neural circuits in the blind on diffusion direction analysis. Neuroreport. 18:1757–1760.
Parker SW, Nelson CA. 2005. An event-related potential study of the impact of institutional rearing on face recognition. Dev Psychopathol. 17:621–639.
Paul R, Henry L, Grieve SM, Guilmette TJ, Niaura R, Bryant R, Bruce S, Williams LM, Richard CC, Cohen RA, et al. 2008. The relationship between early life stress and microstructural integrity of the corpus callosum in a non-clinical population. Neuropsychiatr Dis Treat. 4:193–201.
Pryce CR, Feldon J. 2003. Long-term neurobehavioural impact of the postnatal environment in rats: manipulations, effects and mediating mechanisms. Neurosci Biobehav Rev. 27:57–71.
Rolls ET. 2004. The functions of the orbitofrontal cortex. Brain Cogn. 55:11–29.
Roosendaal SD, Geurts JJ, Vrenken H, Hulst HE, Cover KS, Castelijns JA, Pouwels PJ, Barkhof F. 2009. Regional DTI differences in multiple sclerosis patients. NeuroImage. 44:1397–1403.
Rutter ML, Kreppner JM, O’Connor TG. 2001. Specificity and heterogeneity in children’s responses to profound institutional privation. Br J Psychiatry. 179:97–103.
Sattler J. 2001. Assessment of children: behavioral applications. La Mesa, CA: Sattler Publications.
Schmahl C, Bremner JD. 2006. Neuroimaging in borderline personality disorder. J Psychiatr Res. 40:419–427.
Schmahmann JD. 2006. Fiber pathway of the brain. New York: Oxford Univesity Press.
Schmahmann JD, Pandya DN, Wang R, Dai G, D’Arceuil HE, de Crespigny AJ, Wedeen VJ. 2007. Association fibre pathways of the brain: parallel observations from diffusion spectrum imaging and autoradiography. Brain. 130:630–653.
Schneider JF, Il’yasov KA, Hennig J, Martin E. 2004. Fast quantitative diffusion-tensor imaging of cerebral white matter from the neonatal period to adolescence. Neuroradiology. 46:258–266.
Sharma A, Dorman MF, Kral A. 2005. The influence of a sensitive period on central auditory development in children with unilateral and bilateral cochlear implants. Hear Res. 203:134–143.
Shimony JS, Burton H, Epstein AA, McLaren DG, Sun SW, Snyder AZ. 2006. Diffusion tensor imaging reveals white matter reorganization in early blind humans. Cereb Cortex. 16:1653–1661.
Shu N, Li J, Li K, Yu C, Jiang T. 2009. Abnormal diffusion of cerebral white matter in early blindness. Hum Brain Mapp. 30:220–227.
Smith SM, Jenkinson M, Johansen-Berg H, Rueckert D, Nichols TE, Mackay CE, Watkins KE, Ciccarelli O, Cader MZ, Matthews PM, et al. 2006. Tract-based spatial statistics: voxelwise analysis of multisubject diffusion data. NeuroImage. 31:1487–1505.
Sonuga-Barke EJ, Beckett C, Kreppner J, Castle J, Colvert E, Stevens S, Hawkins A, Rutter M. 2008. Is sub-nutrition necessary for a poor outcome following early institutional deprivation? Dev Med Child Neurol. 50:664–671.
Spadoni AD, McGee CL, Fryer SL, Riley EP. 2007. Neuroimaging and fetal alcohol spectrum disorders. Neurosci Biobehav Rev. 31: 239–245.
Stricker NH, Schweinsburg BC, Delano-Wood L, Wierenga CE, Bangen KJ, Haaland KY, Frank LR, Salmon DP, Bondi MW. 2009. Decreased white matter integrity in late-myelinating fiber pathways in Alzheimer’s disease supports retrogenesis. NeuroImage. 45: 10–16.
Suomi SJ. 1997. Early determinants of behaviour: evidence from primate studies. Brit Med Bull. 53:170–184.
Tottenham NHT, Quinn BT, McCarry TW, Nurse M, Gilhooly T, Milner A, Galvan A, Davidson MC, Eigsti IM, Thomas KM, et al. Forthcoming 2009. Prolonged institutional rearing is associated with atypically larger amygdala volume and difficulties in emotion regulation. Dev Sci.
Van den Bergh BR, Mulder EJ, Mennes M, Glover V. 2005. Antenatal maternal anxiety and stress and the neurobehavioural development of the fetus and child: links and possible mechanisms. A review. Neurosci Biobehav Rev. 29:237–258.
Vandermeeren Y, Bastings E, Good D, Rouiller E, Olivier E. 2003. Plasticity of motor maps in primates: recent advances and therapeutical perspectives. Rev Neurol (Paris). 159:259–275.
Versace A, Almeida JR, Hassel S, Walsh ND, Novelli M, Klein CR, Kupfer DJ, Phillips ML. 2008. Elevated left and reduced right orbitomedial prefrontal fractional anisotropy in adults with bipolar disorder revealed by tract-based spatial statistics. Arch Gen Psychiatry. 65:1041–1052.
Yu JT, Tan L. 2008. Diffusion-weighted magnetic resonance imaging demonstrates parenchymal pathophysiological changes in epilepsy. Brain Res Rev. 59(1):43–41.