Originally Authored by Ajay Kumar, Michael E. Behan, Piti Singsoonsud, Amy L. Veenstra, Courtney Wolfe-Christensen, Emily Helder, Harry T. Chugani in Jounral of Child Neurology
Abstract
This study utilized diffusion tensor imaging fiber tractography to examine the miscrostructural integrity of limbic and paralimbic white matter tracts in thirty-six children (Mean age=124 months) with histories of early deprivation, raised from birth in orphanages and subsequently adopted into the United States, compared to 16 age-matched typically developing children. We found increased mean diffusivity bilaterally in the arcuate fasciculus, and increased mean diffusivity and reduced fractional anisotropy bilaterally in the uncinate fasciculus and cingulum in children with early deprivation. Microstructural integrity of the left arcuate fasciculus and right cingulum was related to language and behavioral functioning, respectively. White matter abnormalities were also associated with length of deprivation and time in the adoptive home. Our findings suggest that white matter pathways, connecting limbic and paralimbic brain regions is abnormal in children with histories of early deprivation; with some pathways appearing more susceptible to early deprivation than others.
Keyword: Orphans; Diffusion tensor imaging; Fiber tractography; Neuroimaging; White-matter
Introduction
Early severe deprivation is often associated with neurocognitive delay and/or impairment.(1,2) It has also been associated with an increased incidence of internalizing and externalizing behavioral problems. (3) Previous studies have demonstrated functional(4–6) and structural abnormalities (7–9) in limbic and paralimbic brain regions in children raised in orphanages and subsequently adopted into families in the United States. However, there has been relatively little empirical investigation of white matter, in general, and individual pathways or fiber bundles, in particular, that connect these identified brain regions. It is now possible to isolate white matter tracts using magnetic resonance diffusion tensor imaging and calculate various diffusion parameters, such as fractional anisotropy and mean diffusivity, which reflect their structural integrity.
Using diffusion tensor imaging tractography, we previously demonstrated abnormality in the uncinate fasciculus in seven children with histories of early deprivation, but not in other limbic pathways, possibly due to the small sample size (n=7 per group).(9) Subsequently, in a larger sample, we found more widespread white matter abnormalities, particularly in the regions of uncinate and superior longitudinal fasciculus, possibly involving the arcuate fasciculus, using tract-based spatial statistics (an objective voxel-based method for whole brain diffusion tensor imaging data analysis).(7) As this approach precluded the evaluation of individual fiber tracts, closer examination of various limbic and paralimbic pathways, putatively subserving those functions most commonly impaired in children with histories of early deprivation (i.e., language, behavioral difficulties), is warranted to better understand and delineate the neuro-anatomic correlates underlying these problems.
Therefore, the current study used MR-diffusion tensor imaging and fiber tractography to evaluate the microstructural integrity of four limbic and paralimbic association tracts, i.e., arcuate and uncinate fasciculi, cingulum and fornix, in children with histories of early deprivation compared to age-matched typically developing children. The study was guided by the following hypotheses: 1) compared to typically developing, nonadopted controls, children in the early deprivation group would evidence reduced integrity (i.e., higher mean diffusivity and/or lower fractional anisotropy) of limbic and paralimbic cortical association tracts; 2) mean diffusivity and/or fractional anisotropy of the arcuate fasciculus would be associated with language functioning; 3) integrity of limbic pathways would be associated with socio-emotional functioning; 4) the groups would not differ on mean diffusivity or fractional anisotropy for the corticospinal tract, a sensory-motor pathway.
Methods
Participants
Thirty-six right-handed children (124 ± 28) months; range 68 to 189 months; 13 males, 23 females), placed in orphanages at birth (Mean duration =26 ± 19) months, range 4 to 81 months) and later adopted into the US were included in the study. All of the children, with the exception of one pair of biological siblings, were singleton adoptions. Participants had resided in their adoptive homes for an average of 94 ± 33 months. The sample included children adopted from the following regions: Northern Asia (n=14), Southern/Eastern Asia (n=10) and Eastern Europe (n=12). Mean body mass index measured at the time of the study were 16.6 ± 3.0, placing the group at approximately the 15th percentile. Mean head circumference t-score, also measured at the time of the study, was 31.5 ± 19. Twenty children were taking psychoactive medication at the time of participation. Adopted mother’s and father’s mean years of education were 16.08 ± 2.0 and 16.1 ± 2.8 years, respectively. All the children had normal structural MRI.
The control group consisted of 16 right-handed, typically developing, non-adopted children (9 males, 7 females; Mean age =127 ± 30) months; range 72 to 186 months). Mean body mass index was 20.9 ± 4.6. Their intellectual functioning was measured to be within normal limits.
Children who evidence the presence of or history of exposure to factors with known neurobiological effects (e.g., alcohol) were excluded from the study. Study procedure, specific inclusion/execution criteria, and excluded participants have been described previously.(2) The study was performed with the approval of and in accordance with the guidelines stipulated by the Human Subjects Research Committee at Wayne State University, Detroit, MI, USA, and written informed consent was obtained from all study participants.
Neuropsychological Evaluation
Children in the early deprivation group completed an extensive neuropsychological assessment, while children in the control group completed a brief neuropsychological battery, as described previously.(2,10)
MR Image Acquisition and Fiber Tractography
MRI scanning was performed using a 1.5 Tesla magnet (Siemens Sonata, Erlangen, Germany). The specific MR-diffusion tensor imaging acquisition and fiber tractography protocols have been described previously.(9,11–13) In brief, the individual fiber tracts (Figure 1) were isolated as follows: For the arcuate fasciculus, a region of interest was placed in the coronal plane at the level of the posterior tip of the putamen using the “OR” operator lateral to the superior aspect of the corona radiata. A second region of interest in the axial plane was then placed using the “AND” operator at the level just below the Sylvian fissure where the arcuate fasciculus can be discretely identified. A two region of interest approach was used to isolate the uncinate fasciculus. The first “OR” region of interest was placed in the frontal lobe at the coronal level corresponding to the posterior tip of the caudate and a second “AND” region of interest was placed in the anterior temporal lobe at the same coronal level as the first region of interest. The diffusion tensor imaging color map was used to isolate Cingulum and Fornix as they can be discretely identified as a green color bundle just above or below the corpus callosum, respectively. Multiple sagittal regions of interest around the visible green color were used to extract them. The corticospinal tract was isolated by drawing one region of interest around the posterior limb of the internal capsule and another region of interest around the CST in the brain stem (identified as a blue color bundle in the anterior part of the brain stem) on the axial slices.
After isolation, fractional anisotropy and mean diffusivity were calculated for each the tracts. Tractography was performed independently by two investigators (AK and PS), who were blind to subject group. Inter-rater reliability was assessed by determining the correlation of fractional anisotropy and mean diffusivity values obtained between observers; the overall correlation coefficient of the measurements was 0.94 (p<0.01).
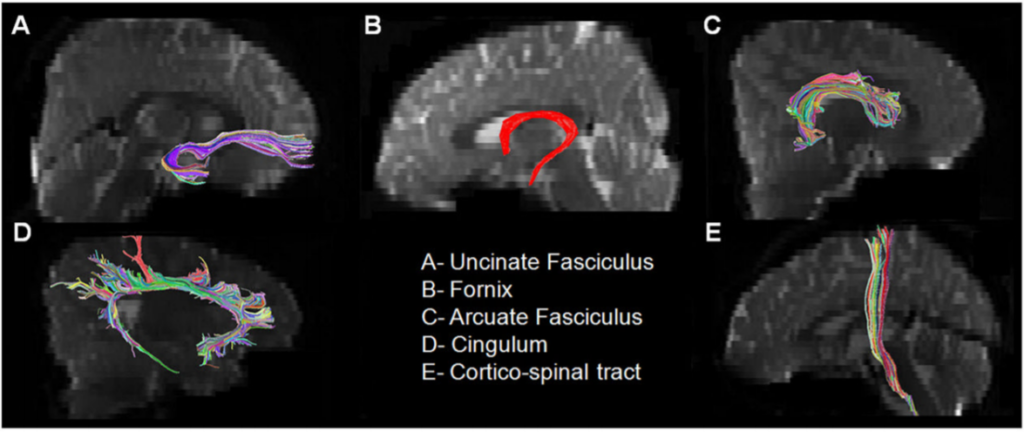
Corticospinal tract.
Statistical Analysis
Values are given as mean standard deviation, unless noted otherwise. Preliminary analyses involved correlations between age, gender, body mass index, parent education, medication use, and diffusion tensor imaging metrics (fractional anisotropy, mean diffusivity) for each of the tracts. Variables correlated with outcome measures were included in subsequent analyses as covariates.
The hypothesis that the early deprivation group would show reduced integrity of white matter tracts was tested via separate 2 (Group) × 2 (Side) repeated measures analysis of variance with mean diffusivity and fractional anisotropy for each tract as variables. When the overall test was significant, follow-up analyses were performed to determine the nature of the group differences. Since the right side of the arcuate fasciculus is often not wellidentified using deterministic tractography, separate univariate analysis of co-variance, for the left and right hemisphere, with group as the between subjects factor and mean diffusivity and fractional anisotropy as the outcomes, were performed.
Associations between orphanage-specific variables (region of institution, duration of orphanage experience, duration in the adoptive home), language (total language standard score), and behavioral functioning (internalizing and externalizing problems) and diffusion tensor imaging metrics (mean diffusivity and fractional anisotropy for each tract) were investigated using partial correlations. For region of institution, separate analysis of covariance for mean diffusivity and fractional anisotropy were used. To reduce the number of comparisons only theoretically indicated correlations were examined. Specifically, mean diffusivity and fractional anisotropy for the arcuate fasciculus were correlated with total language score; mean diffusivity and fractional anisotropy for cingulum, uncinate fasciculus, and fornix were correlated with externalizing and internalizing behavioral scores. Despite this design, a total of 52 correlations were conducted; therefore, in order to provide statistical control for multiple comparisons an alpha level of 0.01 was used to determine significance.
Results
Age was negatively correlated with mean diffusivity values in bilateral uncinate fasiculi (left, p=0.002; right, p=0.017), bilateral cingulum (left, p=0.000; right, p=0.000), and left arcuate fasciculus (p=0.021). Body mass index was negatively correlated with mean diffusivity values in bilateral cingulum (left, p=0.005; right, p=0.013). Fractional anisotropy values in bilateral cingulum were positively corrected with age (left, p=0.010; right, p=0.000) and body mass index (left, p=0.035; right, p=0.036). Body mass index was also negatively correlated with fractional anisotropy in the left corticospinal tract (p=0.000). Neither gender nor medication was associated with any of the diffusion tensor imaging metrics. The parameters having significant correlations with outcome were subsequently included as covariates in respective analyses.
Neuropsychological Profile
The early deprivation group was functioning within normal limits across neurocognitive domains, with most domains measured within the average range (Table 1). Relative (to full scale intelligence quotient) deficits in functioning were noted in verbal memory (Standard Score=83.43) and impulsivity (t-score=80.69). Caregiver-report of externalizing behavioral problems revealed a group mean in the “at-risk” range (t-score=61.82).
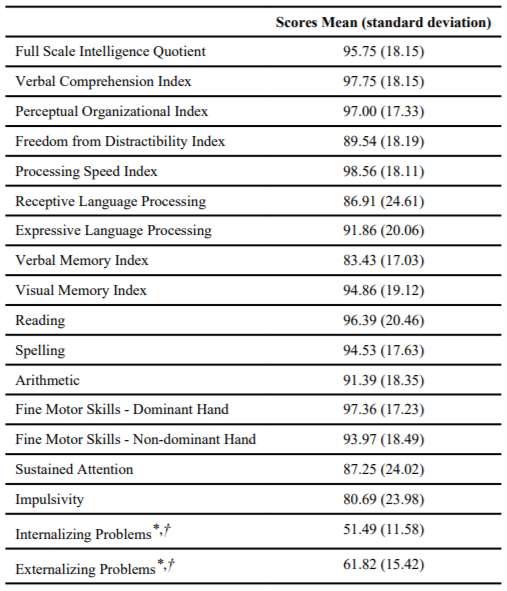
Data are presented in standard scores (mean=100; standard deviation=15) unless otherwise noted as
*t-score (mean=50; standard deviation=10);
†Item measured by “Behavior Assessment System for Children” at the “at risk” range
Fiber Tracking: Between-Group analysis
The left arcuate fasciculus was identifiable in all subjects; however the right arcuate fasciculus was only identified in 62.5% (10/16) of controls and 52.8% (19/36) of the early deprivation group. The groups did not significantly differ in percentage of children with unidentifiable right arcuate fasciculus (Chi-square =0.42, p = 0.52). Tests for between group differences on demographic and neurocognitive variables revealed no significant differences between children in whom the right arcuate fasciculus could or could not be identified.
Table 2 presents the data for fractional anisotropy and mean diffusivity by group and region. The early deprivation group showed reduced fractional anisotropy and increased mean diffusivity for both the left and right arcuate fasciculi as compared to the control group. For fractional anisotropy, the univariate analysis of variance for left arcuate fasciculus was significant (p=0.035). For the right arcuate fasciculus, the test was not significant. For mean diffusivity, univariate tests revealed significantly increased mean diffusivity, bilaterally, for the early deprivation group as compared to controls (left, p=0.000; right, p=0.038).
There was also reduced fractional anisotropy and increased mean diffusivity in the early deprivation group for uncinate fasciculus as compared to controls (Table 2). For fractional anisotropy in uncinate fasciculus, the mixed design analysis of co-variance showed a significant main effect for group (p=0.006). Similarly, there was a significant main effect for group for mean diffusivity (p=0.010), controlling for age.
For the cingulum, separate mixed design analyses of co-variance, controlling for age and body mass index, revealed significant main effects for group for fractional anisotropy (p=0.013) and mean diffusivity (p=0.002). Children with histories of early deprivation had decreased fractional anisotropy and increased mean diffusivity compared to controls.
Although the early deprivation group showed reduced fractional anisotropy and increased mean diffusivity for the fornix, compared to controls, the differences did not reach statistical significance. fractional anisotropy and mean diffusivity for corticospinal tract were essentially similar between early deprivation children and normal control group.
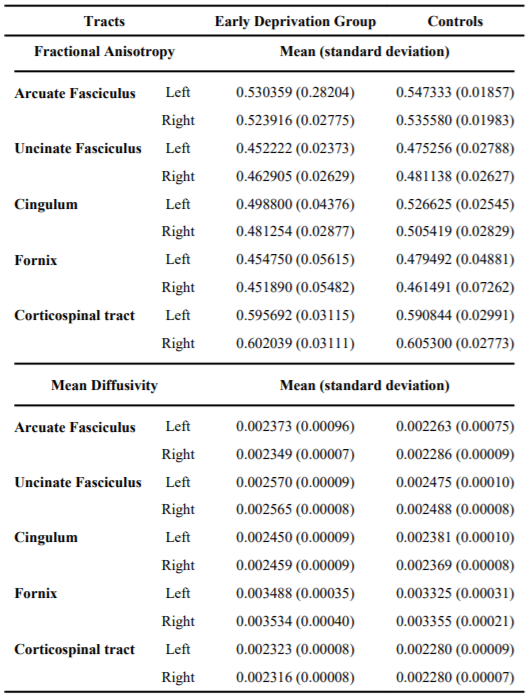
Correlations Between Orphanage-Specific, Neurocognitive, and Behavioral Functions and Fiber Tracking Metrics
Language Functioning. After controlling for age, overall language functioning was significantly positively related to fractional anisotropy for the left arcuate fasciculus (p=0.001, Figure 2), but was unrelated to mean diffusivity.
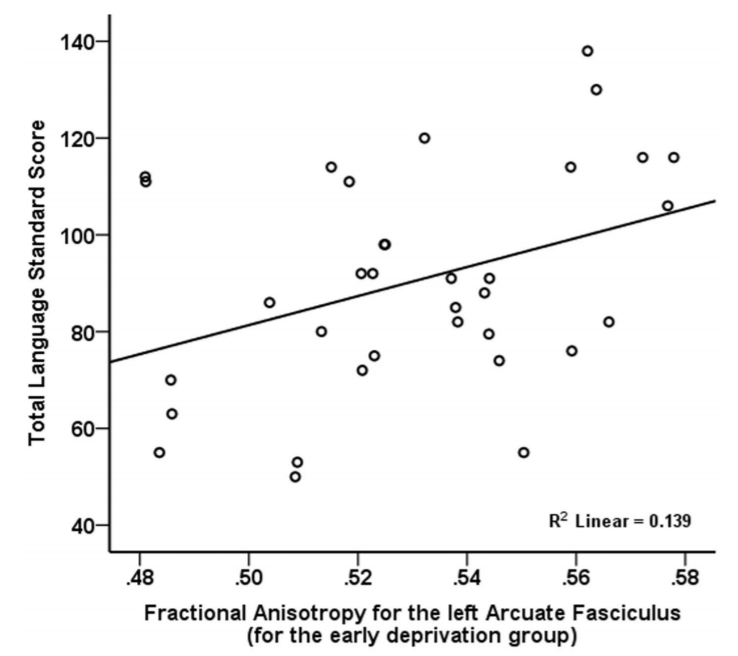
Behavioral Functioning. After controlling for age and body mass index, externalizing problems were significantly negatively correlated with fractional anisotropy in the right cingulum (p=0.004). There was also a trend for a positive association between mean diffusivity in the right cingulum and externalizing behavioral problems (p=0.027). No significant correlations were found between diffusion tensor imaging metrics and internalizing problems.
Orphanage-Specific Variables. Duration of orphanage experience showed nonsignificant trends for an inverse association with fractional anisotropy for the right uncinate fasciculus (p=0.039) and right fornix (p=0.020) but was unrelated to mean diffusivity. Although not related to fractional anisotropy for any of the tracts, length of time in the adoptive home was significantly negatively correlated with mean diffusivity for the left arcuate fasciculus (p=0.006, Figure 3), and left cingulum (p=0.003). Region of adoption was not associated with diffusion tensor imaging metrics.
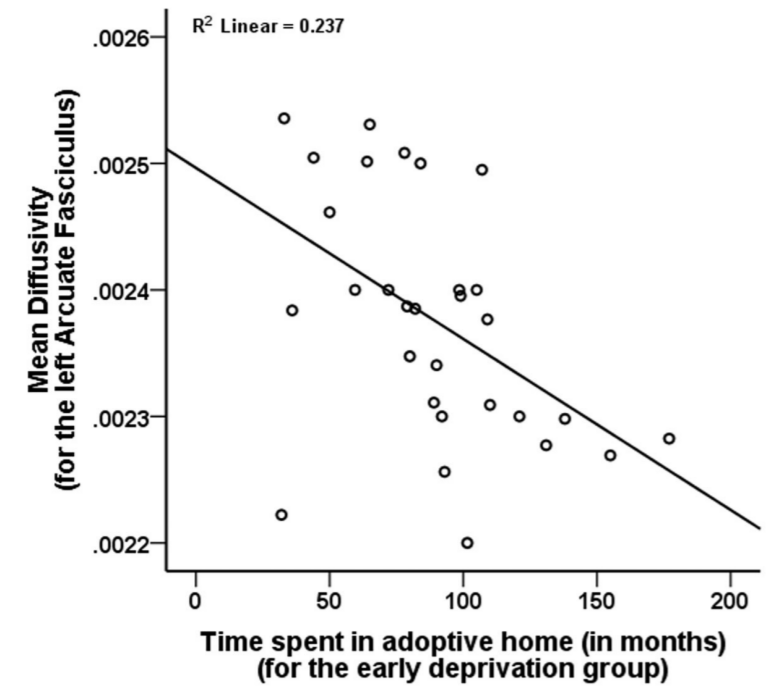
Discussion
In the current study there were significant differences in fractional anisotropy and mean diffusivity for several limbic and paralimbic white matter pathways for children with histories of early deprivation as compared to control children. Additionally, the integrity of these pathways was associated with language, emotional and behavioral functioning. These findings add to the growing body of research showing links between early postnatal deprivation, behavior, and localized abnormalities revealed by advanced functional and structural neuroimaging methodologies. (8)
Mechanisms of Changes in Diffusion Parameters and Their Implications
Diffusion tensor imaging is a very sensitive method to detect white matter maturation and alteration. Abnormalities in myelination, axonal number, diameter and orientation can all lead to changes in fractional anisotropy and mean diffusivity.(14) The relative sensitivity of the various diffusion parameters in identifying abnormalities may depend upon the underlying pathology. For example, a change in diffusion in all directions, such as in brain edema, stroke or cell death, mean diffusivity is likely to be a very sensitive measure as it represents the average of diffusivities along three main directions (x, y and z). Abnormally increased fiber crossing, or different or unusual fiber orientation can also lead to change in mean diffusivity. On the other hand, a change in diffusivity along one particular direction (i.e., along the fibers or perpendicular to it), such as change in myelination or in the case of increased axonal swelling, axonal thinning or increased intercellular space, tends to magnify the change in fractional anisotropy. The proposed mechanisms for decreased fractional anisotropy are loss or lack of myelination,(15) loss of axons, gliosis, and tissue edema. In addition, nascent axon sprouting, axonal spin formation, and architectural variations such as increased axonal crossing, can also result in decreased fractional anisotropy.(16) As the diffusion anisotropy increases, the fractional anisotropy measurement is likely to be the more sensitive parameter in identifying such changes.
Loss of microstructural integrity in various limbic and paralimbic pathways in the current study could have resulted from a developmental or formational abnormality, potentially due to the multifaceted deprivation associated with the orphanage experience, including lack of exposure to experience-expectant stimulation.(17) The notion that lack of exposure to certain stimuli during brain development can result in a loss of microstructural integrity of selective white matter pathways as measured by diffusion tensor imaging is not novel. For example, inadequate exposure to experience-expectant visual stimuli has been shown to reduce fractional anisotropy in the optic radiation in humans suffering from early blindness.(18,19) Numerous other studies have shown that deprivation of sensory, motor, and social stimulation has profound effects on both function and structure of the developing nervous system and in domain-specific systems.(20–23)
Alternatively, the diffusion tensor imaging findings in this study could be secondary to chronic experiences of stress, as social deprivation can result in a dysregulated stressresponse.(24,25) Studies of both animals and humans have documented that prolonged stress impacts both structure and function of the central nervous system;(26–29) with high levels of stress over an extended period of time leading to adverse focal consequences.(30) For example, in humans, stressful life events in childhood are correlated with reduced fractional anisotropy for the genu of the corpus callosum, left arcuate fasciculus, cingulum, and fornix.(31) Interestingly, observed abnormalities for early deprivation children involve regions particularly susceptible to the physiological effects of stress.(8,29) The prolonged stress of inconsistent care may alter biological systems during development, thus playing an important role in producing the abnormalities observed in this study.
Several additional factors may have contributed to reduced microstructural integrity in the early deprivation group. First, poor prenatal and postnatal nutrition have been linked to poor myelination(32) and neurotransmission,(33) and the small physical stature and head circumference in the early deprivation group provide evidence for a history of malnutrition. Secondly, adverse affects on the developing nervous system could result from maternal stress during pregnancy.(34) Furthermore, prenatal exposure to alcohol has been shown to affect both structure and function in the brain,(35) including increased white matter volume.(36)
With limited records of biological mothers’ living conditions and lifestyle, it is possible children with early deprivation in this study were exposed to stressors or toxins in-utero; however neurologic sequelae secondary to a poor or toxic prenatal environment are expected to have global effects(37) and this was not evident in the current sample.
Association of Language and Behavior Functioning with Structural Findings
Children with early deprivation show an increased incidence of language delay and disorder, with a portion of such children having persistent language problems. (38) The relationship of overall language to reduced fractional anisotropy for the arcuate fasciculus in the left hemisphere is consistent with prior research conducted in samples with language deficits (i.e. developmental delay; (39) Autism (40). Reduced language stimulation is typical of orphanage rearing1 and may contribute to altered neural pathways/structures that subserve language functions, resulting in impaired language functioning.
The current findings suggest that the elevated incidence of externalizing behavioral problems in this study is associated with reduced integrity of limbic pathways. Recent work has shown increased mean diffusivity for the cingulum in children with attention deficit hyperactivity disorder. (41) Up to 30% of the children with histories of early deprivation express an Inattentive/Overactive phenotype; abnormalities in this pathway may underlie behavioral dysregulation common to these problems. (1,2,42) Integrity of the cingulum has been linked with internalizing behaviors; lower fractional anisotropy for the cingulum is evident in children with post-traumatic stress (43) and depression.(31) These findings provide additional support that alterations in the developing brain, secondary to early deprivation, may underlie neurocognitive and socio-emotional difficulties commonly observed in children raised in orphanages.
Association of Orphanage-Specific Variables with Structural Findings
Length of institutionalization has been established as a predictor of psychosocial outcomes (1,2) and structural integrity. (8) In the current study there were trends toward longer time in the orphanage being related to reduced organization of white matter limbic pathways. Longer period of time in the adoptive home was significantly related to lower mean diffusivity (i.e., improved integrity) for the bilateral arcuate fasciculi, and bilateral cingulum. Interestingly previous research has not supported a strong link between length of time in the adoptive home and neurocognitive or behavioral outcomes. (2,44) However, recent research has suggested that increased length of time in the adoptive home is associated with improved verbal functions. (38) The current results provide empirical support in humans for improvement in neural structure/integrity as time in a normalized environment increases, which is consistent with previous animal research showing resolution of neural abnormalities secondary to early maternal separation or deprivation following exposure to high level maternal stimulation, (i.e., “stroking”). (27)
Methodological Limitations
Recognized methodological problems in diffusion tensor imaging tractography include the issues of crossing fibers, kissing fibers and reproducibility of tracking fiber tracts with complex geometry. The reproducibility of tracking algorithms to identify specific tracts depends primarily on the type of tracts. For example, it is far less reproducible to track some of the thin limbic tracts (such as stria terminalis) that approach the limit of diffusion tensor imaging resolution compared to major limbic, association or projection tracts. (13) The reproducibility of tracking association and limbic tracts on diffusion tensor imaging has been previously validated by us and other groups. (13,45) Further, the results of the present study are largely consistent with those using different technique or analytical approaches (such as, whole brain voxel based analysis;(7) 2-deoxy-2[18F]fluoro-D-glucose positron emission tomography (5) increasing confidence in the present observations.
Although this study used a community-recruited sample of children with early deprivation, the level of global cognitive functioning and behavioral problems in this sample is comparable to randomly selected samples of children with early deprivation. (1,3) Children in the early deprivation group present with relatively deficient performance on measures of verbal memory and impulsivity. Therefore diffusion findings could be associated more with deficient behavioral control. The addition of a control group with similar neurocognitive functioning (i.e., attention deficit hyperactivity disorder in future studies could distinguish diffusion abnormalities associated with history of deprivation or current behavioral difficulties.
Our study demonstrated that children with histories of early deprivation have reduced microstructural integrity of the arcuate fasciculus, uncinate fasciculus, and cingulum. These findings provide additional support for limbic and paralimbic abnormalities in children with early deprivation. Additionally, relationships were identified between diffusion tensor imaging metrics and neurocognitive variables, such that better structural integrity of specific white matter pathways was associated with better language functions, and fewer externalizing behaviors. Additionally, integrity of several tracts was associated with time in the orphanage and adoptive home. Children with histories of early deprivation are exposed to multiple depriving factors that affect both structural and functional brain development. Future research should focus on identifying functional neural correlates (e.g., functional MRI and neurochemistry) of these structural findings.
Acknowledgments
The work was performed at Children’s Hospital of Michigan, Detroit Medical Center, with a generous grant support from National Institute of Mental Health. A part of this work was presented at 38th annual meeting of the Child Neurology Society, in Louisville, Kentucky, in 2009.
References
1. Rutter ML, Kreppner JM, O’Connor TG. English Romanian Adoptees study team. Specificity and heterogeneity in children’s responses to profound institutional privation. Br J Psychiatry. 2001; 179:97–103. [PubMed: 11483469]
2. Behen ME, Helder E, Rothermel R, Solomon K, Chugani HT. Incidence of specific absolute neurocognitive impairment in globally intact children with histories of early severe deprivation. Child Neuropsychol. 2008; 14:453–469. [PubMed: 18686074]
3. Gunnar MR, van Dulmen MH. International Adoption Project T. Behavior problems in postinstitutionalized internationally adopted children. Dev Psychopathol. 2007; 19:129–148. [PubMed: 17241487]
4. Marshall PJ, Reeb BC, Fox NA, Nelson CA 3rd, Zeanah CH. Effects of early intervention on EEG power and coherence in previously institutionalized children in Romania. Dev Psychopathol. 2008; 20:861–880. [PubMed: 18606035]
5. Chugani HT, Behen ME, Muzik O, Juhasz C, Nagy F, Chugani DC. Local brain functional activity following early deprivation: a study of postinstitutionalized Romanian orphans. Neuroimage. 2001; 14:1290–1301. [PubMed: 11707085]
6. Parker SW, Nelson CA. Bucharest Early Intervention Project Core G. An event-related potential study of the impact of institutional rearing on face recognition. Dev Psychopathol. 2005; 17:621–639. [PubMed: 16262985]
7. Govindan RM, Behen ME, Helder E, Makki MI, Chugani HT. Altered water diffusivity in cortical association tracts in children with early deprivation identified with Tract-Based Spatial Statistics (TBSS). Cereb Cortex. 2010; 20:561–569. [PubMed: 19546156]
8. Tottenham N, Hare TA, Quinn BT, et al. Prolonged institutional rearing is associated with atypically large amygdala volume and difficulties in emotion regulation. Dev Sci. 2010; 13:46–61. [PubMed: 20121862]
9. Eluvathingal TJ, Chugani HT, Behen ME, et al. Abnormal brain connectivity in children after early severe socioemotional deprivation: a diffusion tensor imaging study. Pediatrics. 2006; 117:2093– 2100. [PubMed: 16740852]
10. Behen ME, Muzik O, Saporta AS, et al. Abnormal fronto-striatal connectivity in children with histories of early deprivation: A diffusion tensor imaging study. Brain Imaging Behav. 2009; 3:292–297. [PubMed: 19727404]
11. Basser PJ, Pierpaoli C. A simplified method to measure the diffusion tensor from seven MR images. Magn Reson Med. 1998; 39:928–934. [PubMed: 9621916]
12. Mori S, Crain BJ, Chacko VP, van Zijl PC. Three-dimensional tracking of axonal projections in the brain by magnetic resonance imaging. Ann Neurol. 1999; 45:265–269. [PubMed: 9989633]
13. Mori S, van Zijl PC. Fiber tracking: principles and strategies – a technical review. NMR Biomed. 2002; 15:468–480. [PubMed: 12489096]
14. Basser PJ. Inferring microstructural features and the physiological state of tissues from diffusionweighted images. NMR Biomed. 1995; 8:333–344. [PubMed: 8739270]
15. Jito J, Nakasu S, Ito R, Fukami T, Morikawa S, Inubushi T. Maturational changes in diffusion anisotropy in the rat corpus callosum: comparison with quantitative histological evaluation. J Magn Reson Imaging. 2008; 28:847–854. [PubMed: 18821626]
16. Madler B, Drabycz SA, Kolind SH, Whittall KP, MacKay AL. Is diffusion anisotropy an accurate monitor of myelination? Correlation of multicomponent T2 relaxation and diffusion tensor anisotropy in human brain. Magn Reson Imaging. 2008; 26:874–888. [PubMed: 18524521]
17. Greenough WT, Black JE, Wallace CS. Experience and brain development. Child Dev. 1987; 58:539–559. [PubMed: 3038480]
18. Shu N, Liu Y, Li J, Li Y, Yu C, Jiang T. Altered anatomical network in early blindness revealed by diffusion tensor tractography. PLoS One. 2009; 4:e7228. [PubMed: 19784379]
19. Shimony JS, Burton H, Epstein AA, McLaren DG, Sun SW, Snyder AZ. Diffusion tensor imaging reveals white matter reorganization in early blind humans. Cereb Cortex. 2006; 16:1653–1661. [PubMed: 16400157]
20. Lee EJ, Merwine DK, Mann LB, Grzywacz NM. Ganglion cell densities in normal and dark-reared turtle retinas. Brain Res. 2005; 1060:40–46. [PubMed: 16214118]
21. Nakadate K, Imamura K, Watanabe Y. Effects of monocular deprivation on the expression pattern of alpha-1 and beta-1 adrenergic receptors in the kitten visual cortex. Neurosci Res. 2001; 40:155– 162. [PubMed: 11377754]
22. Sharma A, Dorman MF, Kral A. The influence of a sensitive period on central auditory development in children with unilateral and bilateral cochlear implants. Hear Res. 2005; 203:134– 143. [PubMed: 15855038]
23. Vandermeeren Y, Sebire G, Grandin CB, Thonnard JL, Schlogel X, De Volder AG. Functional reorganization of brain in children affected with congenital hemiplegia: fMRI study. Neuroimage. 2003; 20:289–301. [PubMed: 14527589]
24. Carlson M, Earls F. Psychological and neuroendocrinological sequelae of early social deprivation in institutionalized children in Romania. Ann N Y Acad Sci. 1997; 807:419–428. [PubMed: 9071367]
25. Gunnar MR, Morison SJ, Chisholm K, Schuder M. Salivary cortisol levels in children adopted from romanian orphanages. Dev Psychopathol. 2001; 13:611–628. [PubMed: 11523851]
26. Spinelli S, Chefer S, Suomi SJ, Higley JD, Barr CS, Stein E. Early-life stress induces long-term morphologic changes in primate brain. Arch Gen Psychiatry. 2009; 66:658–665. [PubMed: 19487631]
27. Chatterjee D, Chatterjee-Chakraborty M, Rees S, Cauchi J, de Medeiros CB, Fleming AS. Maternal isolation alters the expression of neural proteins during development: ‘Stroking’ stimulation reverses these effects. Brain Res. 2007; 1158:11–27. [PubMed: 17555725]
28. Mirescu C, Peters JD, Gould E. Early life experience alters response of adult neurogenesis to stress. Nat Neurosci. 2004; 7:841–846. [PubMed: 15273691]
29. Kaufman J, Plotsky PM, Nemeroff CB, Charney DS. Effects of early adverse experiences on brain structure and function: clinical implications. Biol Psychiatry. 2000; 48:778–790. [PubMed: 11063974]
30. Bremner JD, Vermetten E. Neuroanatomical changes associated with pharmacotherapy in posttraumatic stress disorder. Ann N Y Acad Sci. 2004; 1032:154–157. [PubMed: 15677402]
31. Choi J, Jeong B, Rohan ML, Polcari AM, Teicher MH. Preliminary evidence for white matter tract abnormalities in young adults exposed to parental verbal abuse. Biol Psychiatry. 2009; 65:227– 234. [PubMed: 18692174]
32. Ward KL, Tkac I, Jing Y, et al. Gestational and lactational iron deficiency alters the developing striatal metabolome and associated behaviors in young rats. J Nutr. 2007; 137:1043–1049. [PubMed: 17374674]
33. Erikson KM, Jones BC, Beard JL. Iron deficiency alters dopamine transporter functioning in rat striatum. J Nutr. 2000; 130:2831–2837. [PubMed: 11053528]
34. Coe CL, Kramer M, Czeh B, et al. Prenatal stress diminishes neurogenesis in the dentate gyrus of juvenile rhesus monkeys. Biol Psychiatry. 2003; 54:1025–1034. [PubMed: 14625144]
35. Spadoni AD, McGee CL, Fryer SL, Riley EP. Neuroimaging and fetal alcohol spectrum disorders. Neurosci Biobehav Rev. 2007; 31:239–245. [PubMed: 17097730]
36. Miller LC, Chan W, Reece RA, Tirella LG, Pertman A. Child abuse fatalities among internationally adopted children. Child Maltreat. 2007; 12:378–380. [PubMed: 17954943]
37. Derauf C, Kekatpure M, Neyzi N, Lester B, Kosofsky B. Neuroimaging of children following prenatal drug exposure. Semin Cell Dev Biol. 2009; 20:441–454. [PubMed: 19560049]
38. Eigsti IM, Weitzman C, Schuh J, de Marchena A, Casey BJ. Language and cognitive outcomes in internationally adopted children. Dev Psychopathol. 2011; 23:629–646. [PubMed: 23786701]
39. Sundaram SK, Sivaswamy L, Makki MI, Behen ME, Chugani HT. Absence of arcuate fasciculus in children with global developmental delay of unknown etiology: a diffusion tensor imaging study. J Pediatr. 2008; 152:250–255. [PubMed: 18206698]
40. Barnea-Goraly N, Kwon H, Menon V, Eliez S, Lotspeich L, Reiss AL. White matter structure in autism: preliminary evidence from diffusion tensor imaging. Biol Psychiatry. 2004; 55:323–326. [PubMed: 14744477]
41. Pavuluri MN, Yang S, Kamineni K, et al. Diffusion tensor imaging study of white matter fiber tracts in pediatric bipolar disorder and attention-deficit/hyperactivity disorder. Biol Psychiatry. 2009; 65:586–593. [PubMed: 19027102]
42. Stevens SE, Sonuga-Barke EJ, Kreppner JM, et al. Inattention/overactivity following early severe institutional deprivation: presentation and associations in early adolescence. J Abnorm Child Psychol. 2008; 36:385–398. [PubMed: 17965931]
43. Kim SJ, Jeong DU, Sim ME, et al. Asymmetrically altered integrity of cingulum bundle in posttraumatic stress disorder. Neuropsychobiology. 2006; 54:120–125. [PubMed: 17199097]
44. Croft C, Beckett C, Rutter M, et al. Early adolescent outcomes of institutionally-deprived and nondeprived adoptees. II: language as a protective factor and a vulnerable outcome. J Child Psychol Psychiatry. 2007; 48:31–44. [PubMed: 17244268]
45. Kumar A, Sundaram SK, Sivaswamy L, et al. Alterations in frontal lobe tracts and corpus callosum in young children with autism spectrum disorder. Cereb Cortex. 2010; 20:2103–2113. [PubMed: 20019145]